Changes in Regional Cerebral Blood Flow Elicited by Craving Memories in Abstinent Opiate-Dependent Subjects
Abstract
OBJECTIVE: The brain circuitry of opiate craving was investigated with positron emission tomography (PET) imaging of regional cerebral blood flow (rCBF). METHOD: Twelve abstinent opiate-dependent subjects listened to audiotaped autobiographical scripts of an episode of craving and a neutral episode while undergoing a PET scan with the tracer [15O]H2O. Statistical parametric mapping was used to analyze the PET images of rCBF changes. RESULTS: Comparison of the drug-related and neutral stimulus conditions revealed activation of rCBF in the left medial prefrontal and left anterior cingulate cortices and deactivation in the occipital cortex in response to the drug-related stimulus. A further statistical parametric mapping analysis with a subjective rating of craving as a covariate showed a positive association of between craving and rCBF in the left orbitofrontal cortex. CONCLUSIONS: The patterns of cerebral activation reflect the different brain regions mediating the salience of opiate-related stimuli and the subjective experience of craving for opiates.
Drug abuse is a chronic condition often characterized by remissions and relapses. It remains unclear why abstinence can be so difficult to maintain for individuals who were previously dependent. Craving is a term often used by opiate-dependent individuals to explain their relapses to heroin use, but defining this term rigorously in a scientific context has proved difficult (1, 2). Although the term is not explicitly used in ICD-10 or DSM-IV, both systems refer to “a persistent desire” or “strong desire or sense of compulsion” when defining the dependence syndrome.
Cue responsivity or cue exposure has been repeatedly used to study craving. Cue responsivity refers to the ability of situations, drug paraphernalia, and mood states or memories associated with previous drug use to effect the desire to use the drug (3). Responses to such cues can be similar or opposite to drug-induced states or can mimic drug withdrawal and may lead to the subjective experience of craving and/or the reinitiation or perpetuation of drug-seeking behavior (4). Cue responsivity has received much attention, because the phenomenon may offer avenues for relapse prevention (5).
The neural circuits that mediate cue responsivity and craving may be accessible with functional neuroimaging techniques. For example, cocaine-related cues and cocaine craving have been shown to induce changes in regional cerebral activation in diverse brain regions. Brain areas that are reported to show activations consistently across paradigms include the dorsolateral prefrontal cortex (6, 7), anterior cingulate cortex (7, 8), amygdala (6, 8), and orbitofrontal cortex (9, 10). The orbitofrontal cortex is also activated in response to heroin-related cues (11). Animal models of drug dependence have implicated many of these brain regions in the positive reinforcing effects of drugs, the negative reinforcing effects of withdrawal, and the ability of environmental stimuli to become conditioned stimuli for both of these effects (4).
Previously we have shown that craving for opiate drugs, in individuals who were dependent on opiates, can be induced by using a cue exposure paradigm of autobiographical scripts (12), even in individuals who have remained abstinent from opiates for 1 year or more (13). We wished to extend this finding in this study by mapping the neural circuits activated in response to this opiate autobiographical cue-exposure paradigm.
We predicted that in opiate-dependent individuals, opiate-related autobiographical cues would activate brain regions associated with responses to opiate or cocaine cues and craving, i.e., the limbic system, in particular the anterior cingulate cortex, the amygdala, the dorsolateral prefrontal cortex, and the orbitofrontal cortex.
Method
Subjects
Twelve subjects (11 men, one woman; mean age=34.4 years, range=27–45 years) with a history of opiate dependence (mean duration=7 years, range=4 months–20 years) were recruited from drug treatment agencies. All were opiate free, as confirmed by urine testing, at the time of the study. The duration of abstinence from opiates before the study was between 10 days and 3 years (precise data were unavailable for two subjects, the mean for the other 10 subjects was 8.25 months). All subjects were right handed. Although seven subjects had a history of abuse of other drugs, none of those subjects was using other illegal drugs at the time of the study. Their status was confirmed by urine testing. Two subjects were taking selective serotonin reuptake inhibitor antidepressants, and one was taking anticonvulsants. No other psychoactive medication was permitted, but one subject was taking the anticholinergic drug oxybutynin for detrusor instability. This study was approved by the local ethics committees and the Administration of Radioactive Substances Advisory Committee. After a complete description of the study, written informed consent was obtained from the subjects.
Protocol
Subjects recorded two audiotaped scripts before the positron emission tomography (PET) scanning session. Each script lasted 2 minutes and recounted a single specific episode from the subject’s past. One of the two scripts described an episode when the subject experienced a strong craving for opiates; the other described a neutral episode. Subjects were specifically told to focus on describing the feelings, both emotional and visceral, of the opiate craving they experienced during the episode they were recounting. Subjects then underwent a 12-run PET scan of regional cerebral blood flow (rCBF) with the tracer [15O]H2O administered with a slow bolus technique. There was a 10-minute gap between runs; the total scan lasted 2 hours. A brain-dedicated ECAT 953b PET camera (CTI PET Systems, Knoxville, Tenn.) operating in a high-sensitivity three-dimensional mode was used. During each of the 12 runs, the subject listened to one of the two audiotaped scripts. Each drug-related and neutral script was presented six times, in random order. Image acquisition began 90 seconds after the beginning of the script to allow craving to be induced in response to the stimulus. Images were acquired in a single 90-second frame. Radioactivity counts acquired during this time frame predominantly reflect changes in rCBF during the rising phase of radioactivity in the head, which typically lasts 30–45 seconds. As described previously (12), after each run the subjects completed six visual analogue scales measuring craving for heroin, urge to use heroin, happiness, sadness, anxiety, and vividness of the script.
Image Analysis
The PET images of rCBF were analyzed with statistical parametric mapping software SPM99 (Wellcome Department of Cognitive Neurology, University College London). The 12 images for each subject were realigned to a mean image for that subject to partially correct for motion of the subject between scans. The realigned images were normalized to a standardized template [15O]H2O PET image. The resultant realigned normalized images were then smoothed with a Gaussian function at 12 mm full width at half maximum.
Statistical analysis of the images was done by using cognitive subtraction and a correlational analysis. For the cognitive subtraction, a condition comparison was carried out, with the audiotaped scripts (drug-related or neutral) determining the conditions. For the correlational analysis, the craving and urge-to-use visual analogue scale scores were averaged to produce a more normally distributed composite crave/urge scale. This composite crave/urge scale was then used as a covariate of interest to examine areas of rCBF that covaried with this scale. This second analysis included only those subjects who reported craving in response to the cue-exposure stimuli. Four subjects were excluded from this analysis since their crave/urge scale scores did not vary from zero throughout the scanning session. To include those subjects who did not report any craving, i.e., whose visual analogue scale scores did not vary, would contravene the basic assumptions of normal distribution that underlie the statistics in statistical parametric mapping, which uses the general linear model.
In the statistical parametric mapping analyses, time from the start of the first scan was entered as a covariate of no interest to take account of any rCBF changes that were related to nonspecific factors. All covariates were centered around subject means and entered with a subject-specific fit. Global cerebral blood flow effects were removed by an analysis of covariance model that allowed for subject-specific fit. The threshold for statistical significance for all analyses was set at p<0.05 after correction for multiple comparisons for either peak change in rCBF or the size of the activated cluster.
In a final analysis, the contrast, or difference, images for each subject comparing the effects of the two conditions were entered into a second-level analysis to examine the effects of duration of abstinence. The two subjects for whom duration of abstinence was not precisely known were excluded.
Results
Subjective Measures
Craving was induced by the cue-exposure paradigm in eight of the 12 subjects (67%), as shown by changes in the visual analogue scale scores. These eight subjects showed a consistent pattern of higher craving and urge-to-use scores in response to the drug-related script than in response to the neutral script, but this pattern attenuated with repetition of the scripts. Figure 1 shows the results for the composite crave/urge scale for each stimulus repetition for these eight subjects. Because the visual analogue scale scores were not normally distributed, they were analyzed by using exact Wilcoxon signed ranks tests to test for differences between the responses to the neutral and drug-related scripts. The difference between responses to the drug-related and to neutral conditions was significant for each repetition (Wilcoxon signed rank one-tailed tests: T=1, N=8, p<0.01 for the first repetition; T=1, N=6, p<0.03 for the second; T=0, N=8, p<0.005 for the third; T=0, N=8, p<0.005 for the fourth; T=1.5, N=6, p<0.05 for the fifth; T=4, N=7, p<0.05 for the sixth). When data for all 12 subjects were included in the analysis, the differences remained significant (Wilcoxon signed rank one-tailed tests: T=1, N=8, p<0.01 for the first repetition; T=1.5, N=7, p<0.03 for the second; T=0, N=8, p<0.005 for the third; T=0, N=8, p<0.005 for the fourth; T=2, N=7, p<0.05 for the fifth; T=6, N=9, p<0.05 for the sixth), although four subjects consistently rated craving and urge to use at or close to zero throughout the scan procedure. The duration of abstinence from opiates did not predict the craving response to the stimuli, nor did it correlate with the degree of craving induced.
The visual analogue scale scores for vividness were intended to control for differences in the intensity of the drug-related and the neutral scripts. There were no significant differences in the scores between the conditions, except for the fifth repetition (T=0, N=6, p<0.05). The visual analogue scale scores for sadness and anxiety showed a consistent trend toward higher scores in response to the drug-related script than in response to the neutral script, but the difference achieved statistical significance only for the second repetition for the sadness scale (T=0, N=9, p<0.01) and for the first and fourth repetitions for the anxiety scale (T=4, N=9, p<0.05, and T=2.5, N=11, p<0.01, respectively). There were no significant differences in the happiness scale scores between the drug-related and the neutral scripts.
Image Analysis
Analysis of the rCBF images revealed two main results. In the cognitive subtraction of conditions, the scans were divided into drug-related or neutral, as determined by the stimulus script. The contrast between these conditions revealed a single significant cluster of voxels (t score=4.53, cluster size=297 voxels, spatial extent p<0.01, corrected for multiple comparisons) showing an increase in rCBF in an area overlying the left medial prefrontal region and adjacent left anterior cingulate cortex (Talairach coordinates:–10, 46, 24 mm) (14) (Figure 2). The reverse contrast of these two conditions to identify areas of relative decrease rCBF in response to the drug-related stimulus showed significant bilateral decreases in rCBF in the primary visual cortex (Talairach coordinates: ±14, –90, 20 mm; t score=3.99 (right) and 4.02 (left), cluster size=193 (right) and 241 (left) voxels, spatial extent p<0.05, corrected for multiple comparisons) and the right extrastriate cortex (Talairach coordinates: 30, –80, 30 mm, t score=3.96, cluster size=378 voxels, spatial extent p<0.005, corrected for multiple comparisons).
The duration of abstinence showed a significant positive association with the size of the difference in rCBF responses between the two conditions in the region of the left anterior cingulate (Talairach coordinates: –8, 38, 18 mm, t score=7.69, cluster size=10 voxels, peak height p<0.02, spatial extent p<0.001, corrected for multiple comparisons). This finding implies that the longer the duration of abstinence from opiate drugs, the larger the change in rCBF between the conditions (Figure 3).
In the correlational analysis, activation in a single region in the left orbitofrontal cortex (Talairach coordinates: –26, 44, –14 mm) was found to covary positively with the composite crave/urge score (t score=5.19, cluster size=157 voxels, peak height p<0.05, corrected for multiple comparisons) (Figure 4). There were also multiple areas of activation in the occipital cortex that covaried negatively and significantly with the composite crave/urge scores. The most significant area was in the right occipital cortex (Talairach coordinates: 12, –90, 22 mm, t score=5.31, cluster size=890 voxels, spatial extent and peak height p<0.01, corrected for multiple comparisons).
The region of the orbitofrontal cortex with activation that correlated with the crave/urge score did not show a significant activation in the condition comparison. Similarly, the region in the left medial prefrontal and anterior cingulate that showed a significant activation in the condition comparison did not show a significant association between activation and the crave/urge score.
Discussion
Two dissociable activation patterns of regional cerebral activation were observed in this study. The orbitofrontal cortex, which showed activation that correlated with the crave/urge scale score, did not show a significant activation with opiate-related cues, and similarly the left anterior cingulate/medial prefrontal region, which was activated by opiate-related stimuli, did not show activation that correlated significantly with the crave/urge scale score. Because these prefrontal foci do not overlap spatially and were identified in fundamentally different analyses, these results suggest that brain responses to drug-related stimuli per se may invoke different brain circuits from those involved in the craving induced by these stimuli.
We could use different methods of analysis to tease apart this distinction because craving induced by the drug-related stimuli carried over into the neutral scans. Specifically, the cognitive subtraction will be maximally sensitive, and a correlational analysis will be relatively insensitive, to detecting responses to the drug-related stimuli that do not show a carryover effect into the next scan. Conversely, areas of activation that show a slower response and particularly are slow to wear off will not be modeled well by the on/off model used for the condition comparison, but a correlational analysis will be maximally sensitive to this pattern.
The increase in rCBF in the left anterior cingulate/medial prefrontal region was consistent across all the subjects, even those who reported no opiate craving, and the response in the anterior cingulate was related to the duration of abstinence. Anterior cingulate activations are commonly observed in functional mapping studies, with a broad distinction being drawn between activation in caudal “cognitive” areas and rostral “affective” regions (15). In our study, the focus of activation clearly lies within the latter region, and thus the activation is more likely to represent a neural response to the emotionally laden nature of the drug-related stimuli than to represent attentional allocation per se to the auditory stimuli. Activation of the anterior cingulate region in response to drug-related video stimuli has been shown to be modulated by activity in the midbrain in response to heroin administration in opiate-dependent subjects (16). This activation was inferior to the activation reported here but remained within the “affective” region. Activation of the anterior cingulate has been reported with cue-induced cocaine craving (7). However, it is not possible from the published data to further localize the effect.
Activation in this region, as measured by single photon emission computed tomography, has in addition been shown to be inversely correlated with the severity of naltrexone-precipitated opiate withdrawal (17). We observed that several of our patients experienced conditioned withdrawal (e.g., sweating and piloerection) while listening to their craving experiences, although we did not measure these responses. Therefore, an alternative explanation is that the activation of the anterior cingulate, an area that was previously associated with withdrawal and pain, was a manifestation of conditioned withdrawal during the craving manipulation in our patients. However, if this explanation were correct, it would be expected that the difference in responses between the opiate-related and the neutral conditions would decrease as the duration of abstinence increased. In contrast, we found that this difference increased as duration of abstinence increased.
In addition, it could be theorized that exposure to stimuli that provoke craving for opiates should become a less familiar experience as the period of abstinence increases. Therefore, any habituation to such stimuli should gradually be lost, and, thus, they would be more arousing or alerting when encountered. Consequently, it would not be surprising that regions involved in emotional arousal and attention, such as the anterior cingulate/medial prefrontal cortex, would show increased relative activation to opiate-related stimuli with increasing length of abstinence.
Activation in the left orbitofrontal cortex showed a positive association with the composite crave/urge scale. This association of rCBF activation was present in all eight subjects who reported a craving response to the stimulus. Activation in this area might be more directly related to the subjective experience of craving rather than the early processing of the opiate-related stimuli. Other researchers have reported a similar significant correlation between urge to use heroin and rCBF in an adjacent area of the left inferior frontal cortex and an area of the orbitofrontal cortex in the opposite, right hemisphere (11). Cocaine craving also correlates with orbitofrontal cortex activation, more medially when precipitated by withdrawal (18) and in the right orbitofrontal cortex when induced by methylphenidate (9). Bilateral orbitofrontal cortex activations are also observed in response to stimuli that elicit cocaine craving (6, 10).
The data we reported here add to the emerging evidence, from studies using nonimaging techniques, that the orbitofrontal cortex plays an important role in drug dependence (19, 20). The orbitofrontal cortex is linked to the mesolimbic dopamine system that mediates reward (21) and to the subcallosal region of the anterior cingulate (19, 20, 22) and is connected to the amygdala. In addition, the orbitofrontal cortex receives inputs from various sensory association areas and consequently plays an important role in expectancy and the reinforcing salience of stimuli (23–25). London et al. (26) have suggested that the orbitofrontal cortex, through its connection with the amygdala, may play a role in evaluating the motivational value of stimuli and labeling the emotional experience of craving. This hypothesis could explain why activation in this region covaried with subjective craving measures in our study. Lastly, Volkow and Fowler (27) have emphasized the role of the orbitofrontal cortex as part of the striatothalamic-orbitofrontal cortex circuit. Dysfunction in this region is associated with compulsive behavior and heightened motivation to access a drug of abuse (27).
The limitations of our study include the lack of comparison subjects and a random effects statistical design. Thus, our conclusions are limited to this particular group of subjects. Only 67% of the subjects reported craving in response to the drug-related stimulus, although this low rate would not be unusual in the artificial environment of a brain scanner. A weakness of our initial categorical design was the carryover effect of craving into the neutral condition. However, we were able to take advantage of this effect by performing a correlational analysis in those subjects who craved to show a different pattern of activation.
The relationship of the visual analogue scale reports of subjective craving to real behavioral intentions is also open to some question, as none of the subjects acted on this craving by relapsing to heroin use. However, the majority of them were seeking help with craving as part of their ongoing treatment, and so the experience of craving was well known to them. The gradual habituation to the craving stimuli, shown by the visual analogue scale scores, in some of the subjects may also have increased the variance of responses, reducing the sensitivity of the cognitive subtraction analysis.
There were minor significant differences in the vividness, anxiety, and sadness visual analogue scales between the two conditions, but it is unlikely that these were responsible for the activations reported, as no significant rCBF changes correlated with scores on these scales (data not shown). Finally, movement by subjects is a possible source of artifactual changes in rCBF. However, for the changes reported here, this explanation is unlikely, because the areas of rCBF change that correlated with subject movement in a separate analysis did not overlap with any of the reported results (data not shown).
In conclusion, we have shown in this study that it is possible to induce opiate craving in a scanner environment and to map the neural correlates of craving and urge to use to activation of the orbitofrontal cortex. This neural response could be distinguished from activations in the left anterior cingulate/medial prefrontal region after exposure to drug-related stimuli.
Received July 7, 2000; revisions received Dec. 28, 2000, and Feb. 20, 2001; accepted Feb. 26, 2001. From the Psychopharmacology Unit, University of Bristol; Medical Research Council Clinical Sciences Centre, Hammersmith Hospital, London; Bristol Specialist Drug Service, Blackberry Hill Hospital, Bristol, U.K.; Avon & Western Wiltshire Mental Health National Health Service Trust, Devizes, U.K.; and Stapleford Centre, Belgravia, London.Address reprint requests to Prof. Nutt, Psychopharmacology Unit, School of Medical Sciences, University of Bristol, University Walk, Bristol, BS8 1TD, UK. Funded in part by a Medical Research Council Programme Grant. Dr. Malizia was supported by a Wellcome training fellowship. The authors thank the staff of the drug treatment agencies, particularly Steve Barnes, for help in recruiting the subjects for this study, and Dr. Dimitris Fouskakis, University of Bristol, for assistance with statistics.
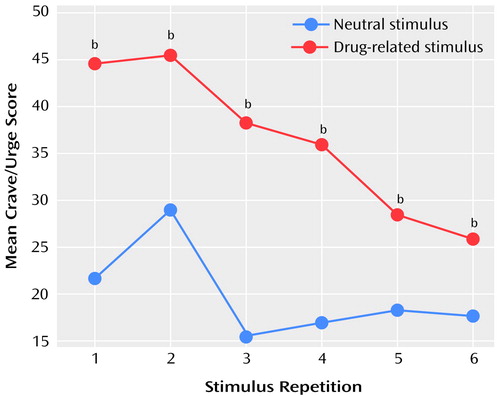
Figure 1. Score on a Composite Crave/Urge Visual Analogue Scalea During Six Repetitions of Audiotaped Drug-Related and Neutral Scripts for Eight Abstinent Opiate-Dependent Subjects Who Reported Craving for Opiates in Response to the Drug-Related Script
aAverage of scores on visual analogue scales measuring level of craving for heroin and urge to use heroin.
bSignificant difference between neutral and drug-related stimuli (Wilcoxon signed rank one-tailed test: T=0–4, N=6–8, p<0.05).
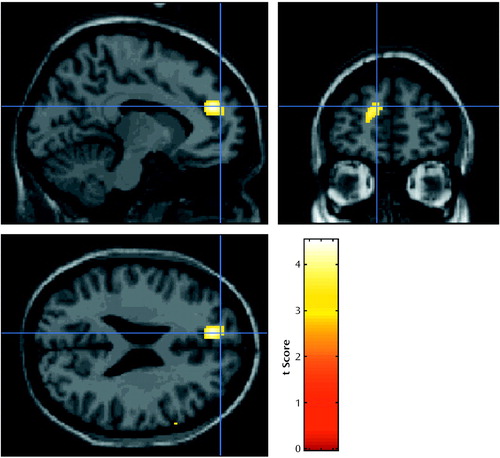
Figure 2. Images Showing Differences in Activation of the Left Medial Prefrontal and Anterior Cingulate Cortex Between Response to a Drug-Related Audiotaped Script and to a Neutral Audiotaped Script in 12 Abstinent Opiate-Dependent Subjectsa
aImages overlaid onto an MRI template. Threshold for display of activation, t=3.18.
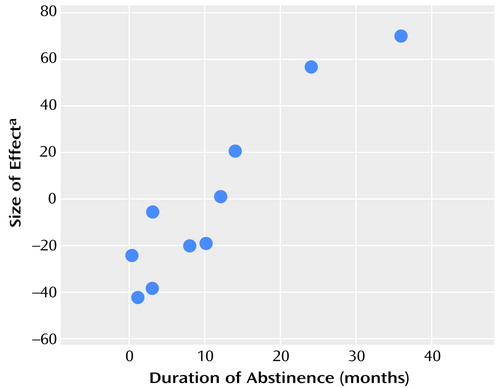
Figure 3. Relation of the Duration of Abstinence From Opiates to the Magnitude of the Difference in rCBF Between Response to a Drug-Related Audiotaped Script and a Neutral Audiotaped Script in 10 Abstinent Opiate-Dependent Subjects
aArbitrary mean-centered units.
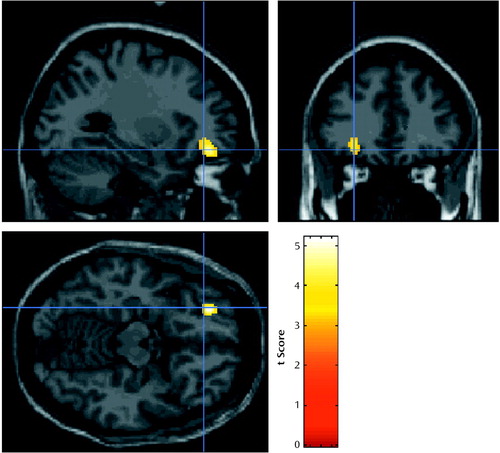
Figure 4. Images Showing Areas of Activation in the Left Orbitofrontal Cortex Correlated With Scores on a Composite Crave/Urge Visual Analogue Scalea for Eight Abstinent Opiate-Dependent Subjectsb
aAverage of scores on visual analogue scales measuring level of craving for heroin and urge to use heroin.
bImages overlaid onto an MRI template. Threshold for display of activation, t=3.18.
1. Robinson TE, Berridge KC: The neural basis of drug craving: an incentive-sensitization theory of addiction. Brain Res Brain Res Rev 1993; 18:247-291Crossref, Medline, Google Scholar
2. Pickens RW, Johanson CE: Craving: consensus of status and agenda for future research. Drug Alcohol Depend 1992; 30:127-131Crossref, Medline, Google Scholar
3. Childress AR, McLellan AT, Ehrman R, O’Brien CP: Classically conditioned responses in opioid and cocaine dependence: a role in relapse? NIDA Res Monogr 1988; 84:25-43Medline, Google Scholar
4. Koob GF: Neurobiology of addiction: toward the development of new therapies. Ann NY Acad Sci 2000; 909:170-185Crossref, Medline, Google Scholar
5. Dawe S, Powell J, Richards D, Gossop M, Marks I, Strang J, Gray JA: Does post-withdrawal cue exposure improve outcome in opiate addiction? a controlled trial. Addiction 1993; 88:1233-1245Crossref, Medline, Google Scholar
6. Grant S, London ED, Newlin DB, Villemagne VL, Liu X, Contoreggi C, Phillips RL, Kimes AS, Margolin A: Activation of memory circuits during cue-elicited cocaine craving. Proc Natl Acad Sci USA 1996; 93:12040-12045Crossref, Medline, Google Scholar
7. Maas LC, Lukas SE, Kaufman MJ, Weiss RD, Daniels SL, Rogers VW, Kukes TJ, Renshaw PF: Functional magnetic resonance imaging of human brain activation during cue-induced cocaine craving. Am J Psychiatry 1998; 155:124-126Link, Google Scholar
8. Childress AR, Mozley PD, McElgin W, Fitzgerald J, Reivich M, O’Brien CP: Limbic activation during cue-induced cocaine craving. Am J Psychiatry 1999; 156:11-18Link, Google Scholar
9. Volkow ND, Wang G-J, Fowler JS, Hitzemann R, Angrist B, Gatley SJ, Logan J, Ding Y-S, Pappas N: Association of methylphenidate-induced craving with changes in right striato-orbitofrontal metabolism in cocaine abusers: implications in addiction. Am J Psychiatry 1999; 156:19-26Link, Google Scholar
10. Wang GJ, Volkow ND, Fowler JS, Cervany P, Hitzemann RJ, Pappas NR, Wong CT, Felder C: Regional brain metabolic activation during craving elicited by recall of previous drug experiences. Life Sci 1999; 64:775-784Crossref, Medline, Google Scholar
11. Sell LA, Morris JS, Bearn J, Frackowiak RS, Friston KJ, Dolan RJ: Neural responses associated with cue evoked emotional states and heroin in opiate addicts. Drug Alcohol Depend 2000; 60:207-216Crossref, Medline, Google Scholar
12. Weinstein A, Wilson S, Bailey J, Myles J, Nutt D: Imagery of craving in opiate addicts undergoing detoxification. Drug Alcohol Depend 1997; 48:25-31Crossref, Medline, Google Scholar
13. Weinstein A, Feldtkeller B, Malizia A, Wilson S, Bailey, Nutt DJ: Integrating the cognitive and physiological aspects of craving. J Psychopharmacol 1998; 12:31-38Crossref, Medline, Google Scholar
14. Talairach J, Tournoux P: Co-Planar Stereotaxic Atlas of the Human Brain: Three-Dimensional Proportional System. Stuttgart, Germany, Georg Thieme, 1988Google Scholar
15. Bush G, Whalen PJ, Rosen BR, Jenike MA, McInerney SC, Rauch SL: The Counting Stroop: an interference task specialized for functional neuroimaging—validation study with functional MRI. Hum Brain Mapp 1998; 6:270-282Crossref, Medline, Google Scholar
16. Sell LA, Morris J, Bearn J, Frackowiak RS, Friston KJ, Dolan RJ: Activation of reward circuitry in human opiate addicts. Eur J Neurosci 1999; 11:1042-1048Crossref, Medline, Google Scholar
17. van Dyck CH, Rosen MI, Thomas HM, McMahon TJ, Wallace EA, O’Connor PG, Sullivan M, Krystal JH, Hoffer PB, Woods SW, Kosten TR: SPECT regional cerebral blood flow alterations in naltrexone-precipitated withdrawal from buprenorphine. Psychiatry Res 1994; 55:181-191Crossref, Medline, Google Scholar
18. Volkow ND, Fowler JS, Wolf AP, Hitzemann R, Dewey S, Bendriem B, Alpert R, Hoff A: Changes in brain glucose metabolism in cocaine dependence and withdrawal. Am J Psychiatry 1991; 148:621-626Link, Google Scholar
19. Di Chiara G, North RA: Neurobiology of opiate abuse. Trends Pharmacol Sci 1992; 13:185-193Crossref, Medline, Google Scholar
20. Wise RA: Neurobiology of addiction. Curr Opin Neurobiol 1996; 6:243-251Crossref, Medline, Google Scholar
21. Koob GF: Drugs of abuse: anatomy, pharmacology and function of reward pathways. Trends Pharmacol Sci 1992; 13:177-184Crossref, Medline, Google Scholar
22. Koski L, Paus T: Functional connectivity of the anterior cingulate cortex within the human frontal lobe: a brain-mapping meta-analysis. Exp Brain Res 2000; 133:55-65Crossref, Medline, Google Scholar
23. Hugdahl K, Berardi A, Thompson WL, Kosslyn SM, Macy R, Baker DP, Alpert NM, LeDoux JE: Brain mechanisms in human classical conditioning: a PET blood flow study. Neuroreport 1995; 6:1723-1728Crossref, Medline, Google Scholar
24. Rolls ET: The orbitofrontal cortex. Phil Trans R Soc Lond B Biol Sci 1996; 351:1433-1443Crossref, Medline, Google Scholar
25. Thorpe SJ, Rolls ET, Maddison S: The orbitofrontal cortex: neuronal activity in the behaving monkey. Exp Brain Res 1983; 49:93-115Crossref, Medline, Google Scholar
26. London ED, Ernst M, Grant S, Bonson K, Weinstein A: Orbitofrontal cortex and human drug abuse: functional imaging. Cereb Cortex 2000; 10:334-342Crossref, Medline, Google Scholar
27. Volkow ND, Fowler JS: Addiction, a disease of compulsion and drive: involvement of the orbitofrontal cortex. Cereb Cortex 2000; 10:318-325Crossref, Medline, Google Scholar