Quantitative Study of Signal Hyperintensities on T2-Weighted Magnetic Resonance Imaging in Late-Onset Schizophrenia
Abstract
OBJECTIVE: The authors investigated the extent and distribution of signal hyperintensities on T2-weighted magnetic resonance imaging (MRI) of the brains of subjects with late-onset schizophrenia. METHOD: The study group consisted of 25 subjects with DSM-III-R schizophrenia and onset at age 50 or more years (late-onset schizophrenia) matched group-wise with 24 subjects with early-onset schizophrenia and 30 psychiatrically healthy volunteers. The subjects underwent clinical and neuropsychological assessments and MRI scans. Transaxial T2-weighted and proton-density images were analyzed manually for signal hyperintensities in cerebral and cerebellar white matter, the basal ganglia, thalamus, and brainstem, and quantitative measures were obtained. RESULTS: Subjects with late-onset schizophrenia had greater periventricular hyperintensities measured as widths of periventricular rims and frontal and occipital caps than the two comparison groups. Hyperintense signals elsewhere in the white matter and in the basal ganglia and brainstem did not differ between groups, but the late-onset schizophrenia group had more signal hyperintensities in the thalamus than the normal comparison group. Signal hyperintensities in the frontal-subcortical circuit regions, when considered together, did not differ between groups. Periventricular hyperintensities had significant negative correlations with intelligence, memory, and frontal-executive functioning in the total group. CONCLUSIONS: The finding of increased periventricular hyperintensities and thalamic signal hyperintensities in late-onset schizophrenia suggests the possibility that cerebrovascular disease, in an interaction with some incompletely understood vulnerability factors, may play a role in the pathogenesis of schizophrenia with onset in advanced age.
Although typically a disorder of young adulthood, schizophrenia is recognized to sometimes develop for the first time in the elderly. Since old age is associated with an increased prevalence of cerebral disorders, a neurological basis for late-onset schizophrenia has long been suspected (1)—i.e., late-onset schizophrenia may in fact be a secondary mental syndrome. Various methods to demonstrate neurological disease in late-onset schizophrenia have included neurological examination for “soft signs,” neuropsychological assessment, and neuroimaging.
Neuroimaging has been the focus of many published studies on late-onset schizophrenia. Computerized tomographic studies have reported lateral ventricular dilatation, atrophy, and the presence of cerebrovascular disease (2–5). Some magnetic resonance imaging (MRI) studies have suggested an excess of white matter lesions in subjects with late-onset schizophrenia in relation to normal comparison subjects, especially on T2-weighted imaging (6–9), but more recent systematic studies have not been supportive of this (10, 11). Signal hyperintensities on T2-weighted imaging are of particular interest because they may indicate the presence of a subtle neurological disease that may not be obvious on physical examination and yet may underpin the development of schizophrenic illness in some individuals. Previous research in this field has been deficient in that signal hyperintensities have been examined qualitatively or quantified by using visual ratings, and the results of systematic quantitation of these lesions in carefully selected patients have not been published. Furthermore, the possibility that it may not be the number or size but the strategic location of these abnormalities in the brain that may be relevant to their relationship to schizophrenia has not been investigated.
We report the results of the quantitative assessment of signal hyperintensities in a group of subjects with late-onset schizophrenia and compare them with two groups matched for age and gender—an early-onset schizophrenia group and a nonpsychiatric comparison group of community volunteers with no neurological disorder. We hypothesized that subjects with late-onset schizophrenia would have an excess of signal hyperintensities in comparison with subjects with early-onset schizophrenia and normal comparison subjects. These signal hyperintensities were predicted to be present particularly in the frontal white matter and the deep nuclei (striatum and thalamus) regions of the brain that constitute the frontal-subcortical neuronal circuits, which are considered to be important in the pathogenesis of schizophrenic disorder.
METHOD
Subjects
Convenience groups of referred patients with schizophrenia were assessed. The late-onset schizophrenia group consisted of 25 Caucasian subjects with a DSM-III-R diagnosis of schizophrenia and an age at onset of 50 or more years. They were compared with 24 subjects with DSM-III-R-defined schizophrenia and an onset before the age of 30 years (early-onset schizophrenia) and 30 nonpsychiatric unpaid community volunteers matched with the subjects with late-onset schizophrenia. The three groups were matched group-wise on gender, and the late-onset schizophrenia and normal comparison groups were matched on age as well. The onset age of 50 years was used to define late-onset schizophrenia, rather than the 45 years suggested by DSM-III-R, to preferentially include somewhat older subjects in the study and to deal with the difficulty in precisely determining the age at onset in some patients. Despite our efforts to recruit very old subjects with early-onset schizophrenia, this group was younger than the other two. All potential subjects were interviewed separately by two study psychiatrists (P.S. and H.B.), who were required to agree on the DSM-III-R diagnosis and age at onset. For the determination of age at onset, corroboration from an informant was necessary.
The following led to exclusion from the study: 1) a history of DSM-III-R-defined alcohol or drug dependence of 5 or more years’ duration or of any duration if within 5 years of the study; 2) a history or physical evidence of stroke or transient ischemic attack, dementia, epilepsy, Parkinson’s disease, any other central nervous system disorder, head injury with loss of consciousness for more than 30 minutes or with any neurological sequelae, or mental retardation; 3) the presence of tardive dyskinesia of greater than mild severity on the Abnormal Involuntary Movement Scale (AIMS) (12) (to reduce its confounding effects on brain imaging parameters); or 4) current major depression or mania. This study was part of a more comprehensive neuropsychiatric study of late-onset schizophrenia, the clinical findings of which will be reported elsewhere. Twelve subjects (two with late-onset schizophrenia, six with early-onset schizophrenia, and four normal comparison subjects) from the larger study were excluded from this report because MRI scans could not be obtained on these subjects owing to refusal (N=8) or practical constraints (N=4). The excluded subjects were not statistically different from the study subjects on age (mean=67.1 years, SD=9.8) (t=1.22, df=89, p=0.23), gender (10 women) (Fisher’s exact test, p=0.73), and education (9.1 years) (t=0.78, df=89, p=0.44). The study was approved by the ethics committees of the University of New South Wales and the Area Health Services, from which subjects were drawn, and written informed consent was obtained from all subjects after the procedures were explained.
Assessment Procedure
From interviews of subjects and informants and reviews of hospital and medical files, information was systematically gathered on sociodemographic features and illness characteristics. Subjects were rated on the Global Assessment of Functioning Scale (DSM-IV) and the Mini-Mental State examination (13). A full physical assessment was performed, which included a systematic neurological examination for “hard” (14) and “soft” (15) neurological signs, the AIMS (12), the Prince Henry Hospital Akathisia Rating Scale (16), and the Hachinski Ischemic Scale (17). A trained research assistant administered the Scale for the Assessment of Positive Symptoms (18) and the Scale for the Assessment of Negative Symptoms (19).
Brain MRI scans were performed on a 1.5-T superconducting magnet (Signa, General Electric, Milwaukee), with the technician blind to the diagnosis and patient groups running concurrently, with the following protocol: a scout T1-weighted mid-sagittal cut; spatially registered axial proton-density and T2-weighted images, using spin echo (TR=1800 msec, TE=20 and 80 msec), from the base of the brain to the apex, with 5-mm-thick sections and 2.5-mm gaps, parallel to the anterior commissure–posterior commissure line on the basis of the mid-sagittal anatomy reviewed interactively at the time of scanning; relatively T1-weighted 1.5-mm-thick contiguous coronal slices (TE=5, TR=24, number of excitations=2, flip angle of 40°), perpendicular to the anterior commissure–posterior commissure line, using three-dimensional acquisition to give good gray/white contrast and anatomical resolution. A 256×256 pixel matrix was used with a 24-cm field of view.
The following battery of tests (for attention, memory, frontal lobe function, and visuospatial function) was used: 1) the National Adult Reading Test (20); 2) WAIS-R (21) selected subtests: picture completion, block design, similarities, comprehension, and vocabulary; 3) the mazes subtest of the WISC-R (22); 4) Wechsler Memory Scale—Revised (23) selected subtests: mental control, figural memory, logical memory I and II, verbal paired associates, visual reproduction I and II, and digit span; 5) the Wisconsin Card Sorting Test, 64-card version (24); 6) the Controlled Oral Word Association Test (25); and 7) Annett’s Hand Preference Questionnaire (26). The scans and the neuropsychological assessments were performed within 2 months of each other.
The T2-weighted and proton-density images were used for measurement. All data were transferred to a Sun workstation and analyzed by using the image-processing software ANALYZE (Biomedical Imaging Resource, Mayo Foundation). Using the atlas by Damasio (27) for guidance, a trained operator who was blind to the diagnosis manually delineated the following regions (right and left) on the axial slices: white matter of frontal, temporal, parietal, and occipital lobes (white matter hyperintensities [WMHs]); cerebellum, basal ganglia (caudate, putamen, and globus pallidum), thalamus, brainstem (midbrain, pons, and medulla oblongata). Hyperintensities in each region were identified as those signals that were clearly visualized as of higher signal intensity on both T2-weighted and proton-density images. Matching T1-weighted brain slices were also examined. If a hyperintense T2-weighted lesion had a matching hypointense T1-weighted lesion that was less than 0.5 cm, it was regarded as a probable Virchow-Robin space and excluded from analysis. If it was greater than 0.5 cm with a matching T1-weighted lesion, it was regarded as a completed infarction and again excluded from analysis. The boundary of each such signal hyperintensity was delineated manually on each T2-weighted brain slice to obtain the number of voxels involved, which gave the total volume of the signal hyperintensity (one voxel=1×1×7.5 mm3). The total hyperintense signal volume for each brain region was determined by summing the individual volumes of signal hyperintensities in that region in all the relevant slices. The right and left cerebral, lateral ventricular, and cerebellar hemisphere volumes were also determined by tracing their boundaries on each slice and summing the results. The periventricular hyperintensities (rims and caps) were quantified differently by measuring their greatest thickness on any proton-density slice at the frontal and occipital horns (caps) and the body of the lateral ventricle (rims) on either side. The sum of the rims and caps measures gave the periventricular hyperintensity measure. The boundaries of the periventricular hyperintensities were too indistinct to reliably obtain volumetric measures on them. Proton-density slices rather than T2-weighted images were used for the periventricular hyperintensity measurements because our pilot investigation showed that the proton-density images offered the best delineation of the boundary between the periventricular parenchyma and the ventricle. The method of delineation of the periventricular hyperintensities is presented in figure 1. To determine the interrater reliability of the measurements, 10 randomly selected scans were rated independently by two trained raters. The intraclass correlation between the two raters for the overall signal hyperintensities measure was high (r1=0.76).
Statistical Analyses
All analyses were conducted by using the Statistical Package for the Social Sciences (28). Data violating univariate normality (z tests on skewness and kurtosis, p<0.05) or homogeneity of variance (Levene test, p<0.05) were examined by using Kruskal-Wallis analyses of variance (ANOVAs) followed by Mann-Whitney U tests. Parametric analyses were conducted with ANOVAs and Scheffé contrasts. Bonferroni corrections were applied to all a priori contrasts.
The signal hyperintensity data were normalized for brain size by expressing the signal hyperintensity volumes as a percent of the same-side cerebral hemisphere volume—(hyperintensity volume/[hemisphere volume–ventricle volume])×100—except for cerebellar hyperintensities, which were expressed as a percent of the same-side cerebellar hemisphere volume. The periventricular hyperintensities (rims and caps), which were linear measures, were normalized by expressing them as a percent of the same-side hemispheric width. ANOVAs were performed on the following aggregated variables, each right and left: brainstem (medulla plus pons plus mesencephalon), frontal lobe WMHs, temporal-parietal lobe WMHs, striatum (caudate plus putamen plus globus pallidus), and periventricular (occipital cap plus frontal cap plus lateral ventricle rim) signal hyperintensities. Included in the analysis was a composite variable labeled frontal-subcortical circuit that contained the sum of scores for thalamus, globus pallidus, putamen, caudate, and frontal lobe WMHs for left and right sides. Analyses were performed on both the raw data (actual volumes of signal hyperintensities and widths of periventricular hyperintensities) as well as the normalized data (as proportions of cerebral or cerebellar hemispheric volumes and hemispheric widths, respectively).
Logistic regression was used to assess the prediction of group membership from signal hyperintensities, allowing for age and education as covariates. For this analysis, left and right side data were summed for each aggregated variable and ranked on a four-level ordinal scale. Age was converted into a three-level ordinal scale representing ranges (<65, 65 to 75, and >75 years). Group membership was examined as two contrasts corresponding to late-onset schizophrenia and early-onset schizophrenia combined compared to the normal comparison group, and the late-onset schizophrenia group compared to the early-onset schizophrenia group. A direct model, with all variables entered simultaneously, was used. Finally, relationships between hyperintensity and neuropsychological test data were examined with nonparametric (Spearman) correlations.
RESULTS
Subject Characteristics
The sociodemographic and clinical characteristics of the subjects are summarized in table 1. Despite attempts to match for age and education, the early-onset schizophrenia group was younger than the other two groups, and the normal comparison group was more educated than the two schizophrenia groups. The subjects were well matched on gender ratio. Schizophrenic subjects had lower Mini-Mental State and Global Assessment of Functioning scores than normal comparison subjects.
MRI Data
The cerebral and cerebellar volumes and hemispheric widths did not differ between the groups. The three groups also did not differ on the signal hyperintensities in the white matter of the frontal, temporal-parietal, and occipital lobes; cerebellum and striatum (caudate, putamen, and globus pallidum); and brainstem (midbrain, pons, and medulla oblongata) (table 2). The late-onset schizophrenia group demonstrated more signal hyperintensities in the thalamus, especially on the right side (Mann-Whitney U=233.0, N=25, 30, p=0.001), than the normal comparison group. More subjects with late-onset schizophrenia (44%, N=11) had thalamic signal hyperintensities than did normal comparison subjects (10%, N=3) (χ2=8.16, df=1, p=0.004, Fisher’s exact test) and subjects with early-onset schizophrenia (25%, N=6) (χ2=1.91, df=1, p=0.17, Fisher’s exact test). Regions in the frontal-subcortical circuit (frontal white matter, striatum, pallidum, and thalamus), when examined together, were again not significantly different across groups. There were, however, significant group differences on all measures of periventricular hyperintensities (normalized) by using analysis of variance with age and years of education as covariates: left ventricular rim (F=6.90, df=2, 76, p=0.002), right ventricular rim (F=3.85, df=2, 76, p=0.03), left frontal cap (F=7.43, df=2, 76, p=0.001), right frontal cap (F=5.98, df=2, 76, p=0.006), left occipital cap (F=6.42, df=2, 76, p=0.003), and right occipital cap (F=7.81, df=2, 76, p=0.001). Except for the right ventricular rim, these differences were significant after Bonferroni corrections. All differences were attributable to higher levels of periventricular hyperintensities in the late-onset schizophrenia group than in the early-onset schizophrenia and normal comparison groups (combined right-side periventricular hyperintensities measures, F=6.48, df=2, 76, p=0.003; combined left-side measures, F=8.28, df=2, 76, p=0.001) (table 2). Even though the subjects with early-onset schizophrenia had slightly greater periventricular hyperintensities than the normal comparison subjects, the differences were not significant on any of the periventricular hyperintensity measures after correcting for age and years of education.
Results of the direct logistic regression are presented in table 3. Contrast A represents the increase in odds of being in the late-onset schizophrenia and early-onset schizophrenia groups (combined) compared to the normal comparison group, for a rank increase in each variable, controlling for age and education. Contrast B represents the late-onset schizophrenia group compared to the early-onset schizophrenia group. Odds ratios with confidence intervals excluding 1.00 were significant at the 0.05 level. For contrast A, 83.7% (N=41) of the late-onset and early-onset schizophrenia subjects and 70% (N=21) of the normal comparison subjects were correctly classified by the model (χ2=39.07, df=1, p<0.001). For all subjects, 78.5% (N=62) were classified correctly. Periventricular hyperintensity was the only significant individual contributor when controlling for education and age (odds ratio=5.54, 95% CI=1.6–19.2, β=1.71, p <0.01). For contrast B, 80% (N=20) of the late-onset schizophrenia subjects and 70% (N=21) of the normal comparison subjects were correctly classified by the model (χ2=19.69, df=1, p<0.001) For all subjects, 75.9% (N=60) were classified correctly. Increased periventricular hyperintensity was the only variable that was significant as an individual predictor (odds ratio=5.49, 95% CI=1.6–19.4, β=1.70, p<0.01). The striatum approached significance as an individual contributor (odds ratio=0.30, 95% CI=0.1–1.1, β=1.20, p=0.06).
Neuropsychological Data
Correlations between neuropsychological and MRI data for all subjects together are presented in table 4, by using a two-tailed significance test. Left and right periventricular hyperintensities were related to poorer test performance (p<0.05) on measures of verbal IQ, vocabulary, comprehension, logical memory I, verbal paired associates, and digit span backward. Left-side periventricular hyperintensities were also related to poorer performance on the WAIS-R similarities test. Left and right frontal WMHs were related to poorer performance on the Wechsler Memory Scale—Revised visual reproduction I test and the categories test of Wisconsin Card Sorting Test. Left frontal WMHs were also negatively correlated with the Wechsler Memory Scale—Revised visual reproduction II test. Increased left- and right-side signal hyperintensities in the composite variables for brainstem and circuit were both negatively correlated with scores for the categories test of the Wisconsin Card Sorting Test; left and right circuit signal hyperintensities were also related negatively to the Wechsler Memory Scale—Revised visual reproduction I test. For the striatum, the only relationship was a negative correlation between the Wechsler Memory Scale—Revised mental control test and right-side signal hyperintensities. Temporal-parietal WMHs were not correlated with any neuropsychological measure except for the categories test of the Wisconsin Card Sorting Test.
Cerebrovascular Risk Factors
The late-onset schizophrenia group had a slightly higher score on the Hachinski Ischemic Scale, but an overall test for the three groups failed to reach significance (Kruskal-Wallis ANOVA, F=5.94, df=1, 78, p=0.05). Hypertension was present in 14 (17.7%) of the subjects overall, diabetes mellitus in three (3.8%), and a history of myocardial infarction in one (1.3%)—none of which was significantly different between groups. Whereas only three subjects with late-onset schizophrenia and three normal comparison subjects were judged to be obese, 12 of 24 (50%) of the subjects with early-onset schizophrenia were obese. A majority of the subjects with early-onset schizophrenia (N=20 of 24, 83.3%) were smokers compared to eight of 24 (33.3%) of the subjects with late-onset schizophrenia and five of 24 (20.8%) of the normal comparison subjects.
DISCUSSION
Hyperintense signals on T2-weighted imaging are reported to be common in the brains of elderly individuals (29), and we found this to be the case in our schizophrenic and nonschizophrenic groups. In fact, no subject had a complete absence of such signals. Our hypothesis that subjects with late-onset schizophrenia would have an excess of these hyperintensities was partially supported. The excess was in the width of the periventricular rims and caps but not in the discrete hyperintense signals in the white matter, basal ganglia, or brainstem—with the exception of the thalamus. We did not find an excess of such signals in the combined strategic brain regions that we had predicted.
The significance of our finding of increased periventricular hyperintensities in late-onset schizophrenia is somewhat uncertain. Mild periventricular hyperintensities are often not indicative of brain disease because they are seen in healthy individuals with no evidence of neuropsychological impairment (30–32). Extensive and severe periventricular hyperintensities are usually associated with intracerebral pathology, but the finding is nonspecific (29–32). The major mechanism for their development is considered to be trans-ependymal absorption of cerebrospinal fluid, usually associated with normal pressure hydrocephalus, subcortical arteriosclerotic encephalopathy, and perivascular atrophy (32, 33). Our subjects did not have any other features of normal pressure hydrocephalus, and we consider cerebrovascular disease to be the most likely etiology for the lesions. Our subjects with late-onset schizophrenia did not have an increase in the risk factors for stroke that we examined in this study, as indicated by the Hachinski Ischemic Scale score and the rates of obesity and smoking. The early-onset schizophrenia group, in fact, had higher rates of smoking. This does not, however, rule out the possibility that the periventricular hyperintensities seen in the subjects with late-onset schizophrenia were due to cerebrovascular disease because the presence of a high Hachinski Ischemic Scale rating is neither necessary nor sufficient for its presence (34).
Our distinction between periventricular and other white matter hyperintensities has some pathophysiological and neuropathological justification. The deep white matter region, which represents the area of the periventricular hyperintensities, is supplied by the deep perforators of the anterior choroidal and the lenticulostriate branches of the middle cerebral arteries. The centrum semiovale region is supplied by the medullary branches of the superficial middle cerebral artery (35). Since the pathophysiology of signal hyperintensities is incompletely understood, this distinction on the basis of vascular supply should arguably be retained, although it is still not clear why. Neuropathologically, the periventricular hyperintensities have been reported to represent demyelination, gliosis, and spongiosis (36, 37). Other signal hyperintensities have been related to the widening of perivascular spaces, perivascular demyelination, vascular ectasia, and cystic and noncystic infarction (30–32, 36, 37). However, irregularly extending periventricular hyperintensities become confluent with WMHs in the centrum semiovale and corona radiata, and demyelination, spongiosis, and infarction may be a common basis for these (38). These lesions may, therefore, represent a continuum of severity of white matter disease, with the mild changes corresponding with subtle impairment (39).
The correlation between signal hyperintensities and neuropsychological impairment has been investigated in many studies. Whereas consistent results have not emerged, most studies (39–43), but not all (44–46), have demonstrated a relationship between the extent of signal hyperintensities and neuropsychological dysfunction. There is a suggestion that there may be a threshold above which these lesions are associated with impairment (41). In our study, some periventricular hyperintensity ratings were significantly correlated with neuropsychological variables. We conclude from this that the periventricular hyperintensities did indicate functional impairment, but the large number of correlations computed for this analysis makes us cautious. The periventricular hyperintensities were particularly associated with deficits in verbal IQ, memory, and frontal-executive functioning—all of which could play a role in the development of psychiatric symptoms. These deficits nevertheless are nonspecific and do not necessarily support the argument for a causal role.
Two studies of signal hyperintensities in late-onset schizophrenia are worthy of detailed comment. The study by Howard et al. (10) compared 38 subjects with late paraphrenia (a broader category than late-onset schizophrenia that includes delusional disorder) with 31 healthy comparison subjects and found no differences in visually rated signal hyperintensities. A quantitative study of white matter hyperintensities in 16 patients with late-onset schizophrenia by Corey-Bloom et al. (33) also reported no differences between early-onset schizophrenia and normal comparison subjects. While methodologically similar to the second study, our study was larger and had more women subjects, which is typical of late-onset schizophrenia, and we examined the signal hyperintensities quantitatively by their anatomical distribution. These methodological differences may account for different results reported by us than in the previous studies.
The finding of higher signal hyperintensities in the thalami of subjects with late-onset schizophrenia than in normal comparison subjects is noteworthy in light of the reports of thalamic abnormality in schizophrenia. Decreased thalamic size has been consistently reported in the early-onset schizophrenia literature—both from neuroimaging (47, 48) and neuropathological (49) studies. Positron emission tomography studies that imaged thalamic metabolism in schizophrenia have been inconsistent (48). The only such study of late-onset schizophrenia (33), on the other hand, reported increased thalamic volumes. The significance of our finding is therefore difficult to ascertain. Moreover, some of our subjects with late-onset schizophrenia had thalamic signal hyperintensities (N=11 of 25), and they did not differ statistically from the subjects with early-onset schizophrenia, raising some doubts about the significance of this finding.
Since the abnormalities demonstrated in our study are quantitative rather than qualitative, they cannot be considered to be specific to late-onset schizophrenia. It is, however, possible that the pathology becomes significant when present in an individual who is vulnerable to the development of schizophrenia. There is evidence from family studies, the assessment of premorbid personality, and the presence of sensory impairment (50–52) that individuals with late-onset schizophrenia are predisposed to the development of psychosis. Our unpublished observations on the same subjects point to a similar conclusion. The basis of this vulnerability is not understood, but in spite of it, these individuals have usually functioned quite well until a late age. It is perhaps the occurrence of additional brain pathology that finally leads to the manifestation of the illness to which they are predisposed. The excess periventricular hyperintensities seen in our subjects with late-onset schizophrenia may be indicative of that additive brain insult. We therefore argue that late-onset schizophrenia is multiply determined, with acquired brain disease, possibly cerebrovascular in origin, interacting with a predisposing abnormality to result in the manifestation of the illness. Since cerebrovascular disease is at least partially preventable, attention to risk factors should lead to a reduction in the incidence of late-onset schizophrenia. Cerebrovascular disease is also likely to progress in many instances so that the subtle cognitive deficits in late-onset schizophrenia may sometimes be progressive. A testable hypothesis to emerge from this study is that a significantly higher proportion of subjects with late-onset schizophrenia will progress to vascular dementia if followed up over a sufficiently long period.
Received Oct. 30, 1998; revision received April 5, 1999; accepted April 12, 1999. From the School of Psychiatry, the University of New South Wales and the Neuropsychiatric Institute, Prince Henry and Prince of Wales Hospitals, Sydney, Australia. Send correspondence to Dr. Sachdev, N.P.I., The Prince of Wales Hospital, Randwick N.S.W. 2031, Australia; [email protected] (e-mail). Supported by grants from the National Health and Medical Research Council of Australia and the Rebecca Cooper Foundation. The authors thank Noelene Rose, Stuart Cathcart, and Ahmad Aniss for help in data collection and analysis, the Mayo Foundation (Dr. Richard Robb and colleagues) for permission to use ANALYZE, Drs. Ron Shnier and Peter Kitchener for neuroradiology opinions, Jeff Looi and Julian Trollor for comments on the article, and Wanda Schinke for secretarial assistance.
![]() |
![]() |
![]() |
![]() |
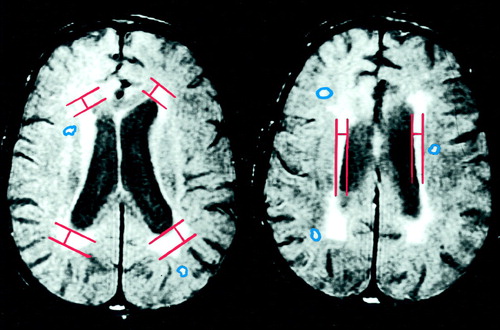
Figure 1. Two Proton-Density Transaxial MRI Brain Slices From a Subject With Late-Onset Schizophrenia That Illustrate the Quantification of Periventricular Hyperintensitiesa
aCaps on left and rims on right. Other white matter hyperintensities were manually delineated on T2-weighted images.
1. Kleist K: Die Involutions-paranoia. Allegemeine Zeitschrift für Psychiatrie 1913; 70:1–64Google Scholar
2. Naguib M, Levy R: Late paraphrenia: neuropsychological impairment and structural brain abnormalities on computed tomography. Int J Geriatr Psychiatry 1987; 2:83–90Crossref, Google Scholar
3. Rabins P, Pearlson G, Jayaram G, Steele C, Tune L: Increased ventricle-to-brain ratio in late-onset schizophrenia. Am J Psychiatry 1987; 144:1216–1218Google Scholar
4. Burns A, Carrick J, Ames D, Naguib M, Levy R: The cerebral cortical appearance in late paraphrenia. Int J Geriatr Psychiatry 1989; 4:31–34Crossref, Google Scholar
5. Howard R, Forstl H, Naguib M, Burns A, Levy R: First-rank symptoms of Schneider in late paraphrenia. Br J Psychiatry 1992; 160:108–109Crossref, Medline, Google Scholar
6. Breitner JCS, Husain MM, Figiel GS, Krishnan KRR, Boyko OB: Cerebral white matter disease in late-onset paranoid psychoses. Biol Psychiatry 1990; 28:266–274Crossref, Medline, Google Scholar
7. Miller BL, Lesser IM, Boone K, Hill E, Mehringer CM, Wong K: Brain lesions and cognitive function in late-life psychosis. Br J Psychiatry 1991; 158:76–82Crossref, Medline, Google Scholar
8. Miller BL, Lesser IM, Mena I, Villanueva-Meyer J, Hill-Gutierez E, Boone K, Mehringer CM: Regional cerebral blood flow in late-life-onset psychosis. Neuropsychiatry Neuropsychol Behav Neurol 1992; 5:132–137Google Scholar
9. Krull AJ, Press G, Dupont R, Harris MJ, Jeste DV: Brain imaging in late-onset schizophrenia and related psychoses. Int J Geriatr Psychiatry 1991; 6:651–658Crossref, Google Scholar
10. Howard R, Cox T, Almeida O, Mullen R, Graves P, Reveley A, Levy R: White matter signal hyperintensities in the brains of patients with late paraphrenia and the normal, community-living elderly. Biol Psychiatry 1995; 38:86–91Crossref, Medline, Google Scholar
11. Symonds LL, Olichney JM, Jernigan TL, Corey-Bloom J, Healy JF, Jeste DV: Lack of significant gross structural abnormalities in MRIs of older patients with schizophrenia and related psychoses. J Neuropsychiatry Clin Neurosci 1997; 9:251–258Crossref, Medline, Google Scholar
12. Guy W (ed): ECDEU Assessment Manual for Psychopharmacology: Publication ADM 76-338. Washington, DC, US Department of Health, Education, and Welfare, 1976, pp 534–537Google Scholar
13. Folstein MF, Folstein SE, McHugh PR: “Mini-Mental State”: a practical method for grading the cognitive state of patients for the clinician. J Psychiatr Res 1975; 12:189–198Crossref, Medline, Google Scholar
14. National Institute of Mental Health: Neurological Examination. Psychopharmacol Bull 1988; 24:647–648Google Scholar
15. Rossi A, De Cataldo S, Di Michele V, Manna V, Ceccoli S, Stratta P, Casaccia M: Neurological soft signs in schizophrenia. Br J Psychiatry 1990; 157:735–739Crossref, Medline, Google Scholar
16. Sachdev P: A rating scale for drug-induced akathisia: development, reliability and validity. Biol Psychiatry 1994; 35:263–271Crossref, Medline, Google Scholar
17. Hachinski VC, Iliff LD, Zilkha E, DuBoulay GH, McAllister VL, Marshall J, Russell RWR, Symon L: Cerebral blood flow in dementia. Arch Neurol 1975; 32:632–637Crossref, Medline, Google Scholar
18. Andreasen NC: Scale for the Assessment of Positive Symptoms (SAPS). Iowa City, University of Iowa, 1984Google Scholar
19. Andreasen NC: Scale for the Assessment of Negative Symptoms (SANS). Iowa City, University of Iowa, 1983Google Scholar
20. Nelson HE: The National Adult Reading Test (NART): Test Manual. Windsor, UK, National Foundation for Educational Research-Nelson, 1982Google Scholar
21. Wechsler D: WAIS-R Manual. New York, Psychological Corp, 1981Google Scholar
22. Wechsler D: Wechsler Intelligence Scale for Children, 3rd ed. San Antonio, Tex, Psychological Corp, 1991Google Scholar
23. Wechsler D: Wechsler Memory Scale—Revised Manual. San Antonio, Tex, Psychological Corp, 1987Google Scholar
24. Berg EA: A simple objective treatment for measuring flexibility in thinking. J Gen Psychol 1948; 39:15–22Crossref, Medline, Google Scholar
25. Benton AL, Hamsher K deS: Multilingual Aphasia Examination. Iowa City, Iowa, AJA Associates, 1989Google Scholar
26. Annett M: A classification of hand preference by association analysis. Br J Psychol 1970; 61:303–321Crossref, Medline, Google Scholar
27. Damasio H: Human Brain Anatomy in Computerized Images. New York, Oxford University Press, 1995Google Scholar
28. 1993 Statistical Package for Social Sciences for Windows, Release 6.0. Chicago, SPSS, 1993Google Scholar
29. Zimmerman RD, Fleming LSA, Lee BCP, Saint-Louis LA, Deck MDF: Periventricular hyperintensity as seen by magnetic resonance: prevalence and significance. Am J Radiol 1986; 146:443–450Google Scholar
30. Sze G, Armond SJ, Brant-Zawadzki M, Davis RL, Norman D, Newton T: Foci of MRI signal (pseudo lesions) anterior to the frontal horns: histological correlations of a normal finding. Am J Radiol 1986; 147:331–337Google Scholar
31. Awad IA, Johnson PC, Spetzler RF, Hudak JA: Incidental subcortical lesions identified on magnetic resonance imaging in the elderly, II: postmortem pathological correlations. Stroke 1986; 17:1090–1097Google Scholar
32. Kertesz A, Black SE, Tokar G, Benke T, Carr, T, Nicholson L: Periventricular and subcortical hyperintensities on magnetic resonance imaging: “rims, caps and unidentified bright objects.” Neurology 1988; 45:404–408Google Scholar
33. Corey-Bloom J, Jernigan T, Archibald S, Harris MJ, Jeste DV: Quantitative magnetic resonance imaging of the brain in late-life schizophrenia. Am J Psychiatry 1995; 152:447–449Link, Google Scholar
34. Lis CG, Gaviria M: Vascular dementia, hypertension, and the brain. Neurol Res 1997; 19:471–480Crossref, Medline, Google Scholar
35. Bogousslavsky J, Regli F: Centrum ovale infarcts: subcortical infarction in the superficial territory of the middle cerebral artery. Neurology 1992; 42:1992–1998Google Scholar
36. Drayer BP: Imaging of the aging brain, I: normal findings. Radiology 1988; 166:785–796Crossref, Medline, Google Scholar
37. Grafton ST, Sumi SM, Stimac GK, Alvord EC, Shaw C-M, Nochlin D: Comparisons of postmortem magnetic resonance imaging and neuropathologic findings in the cerebral white matter. Arch Neurol 1991; 48:293–298Crossref, Medline, Google Scholar
38. Englund E, Brun A, Persson B: Correlations between histopathologic white matter changes and proton MR relaxation times in dementia. Alzheimer Dis Assoc Disord 1987; 1:156–170Crossref, Medline, Google Scholar
39. Ylikoski R, Ylikoski A, Erkinjuntti T, Sulkava R, Raininko R, Tilvis R: White matter changes in healthy elderly persons correlate with attention and speed of mental processing. Arch Neurol 1993; 50:818–824Crossref, Medline, Google Scholar
40. Kluger A, Glanutsos J, Leon MJ, George AE: Significance of age-related white matter lesions. Stroke 1988; 19:1054–1055Google Scholar
41. Boone KB, Miller BL, Lesser IM, Mehringer M, Hill-Gutierrez E, Goldberg MA, Berman NG: Neuropsychological correlates of white-matter lesions in healthy elderly subjects. Arch Neurol 1992; 49:549–554Crossref, Medline, Google Scholar
42. Van Swieten JC, Geykes GG, Derix MM, Peeck BM, Ramos LM, van Latum JC, van Gijn J: Hypertension in the elderly is associated with white matter lesions and cognitive decline. Ann Neurol 1991; 30:825–830Crossref, Medline, Google Scholar
43. Schmidt R, Fazekas F, Michael K, Kapellar P, Augustin M, Offenbacher H, Fazekas G, Lechner H: Magnetic resonance imaging cerebral abnormalities and neuropsychologic test performance in elderly hypertensive subjects: a case-control study. Arch Neurol 1995; 52:905–910Crossref, Medline, Google Scholar
44. Fein G, Van Dyke C, Davenport L: Preservation of normal cognitive functioning in elderly subjects with extensive white-matter lesions of long duration. Arch Gen Psychiatry 1990; 47:220–223Crossref, Medline, Google Scholar
45. Bernardin LJ, Rao SM, Haughton VM, Yetkin ZE, Ellington IE: The neuropsychological significance of leukoaraiosis in a hypertensive population. J Clin Exp Neuropsychol 1991; 13:78–79Google Scholar
46. Schmidt R, Fazekas F, Offenbacher H: Magnetic resonance imaging white matter lesions and cognitive impairment in hypertensive individuals. Arch Neurol 1991; 48:417–420Crossref, Medline, Google Scholar
47. Buchsbaum MS, Someya T, Teng CY, Abel L, Chin S, Najafi A, Haier RJ, Wu J, Bunney WE Jr: PET and MRI of the thalamus in never-medicated patients with schizophrenia. Am J Psychiatry 1996; 153:191–199Link, Google Scholar
48. Andreasen NC, Arndt S, Swayze V II, Cizadlo T, Flaum M, O’Leary D, Ehrhardt JC, Yuh WTC: Thalamic abnormalities in schizophrenia visualized through magnetic resonance image averaging. Science 1994; 266:294–298Crossref, Medline, Google Scholar
49. Shapiro RM: Regional neuropathology in schizophrenia. Schizophr Res 1993; 10:187–239Crossref, Medline, Google Scholar
50. Post F: Persistent Persecutory States of the Elderly. Oxford, UK, Pergamon Press, 1966Google Scholar
51. Kay DWK, Roth R: Environmental and hereditary factors in the schizophrenias of old age (“late paraphrenia”) and their bearing on the general problem of causation in schizophrenia. J Ment Sci 1961; 107:649–686Crossref, Medline, Google Scholar
52. Almeida OP, Howard R, Levy R, David A: Psychotic states arising in late life (late paraphrenia): the role of risk factors. Br J Psychiatry 1995; 166:215–222Crossref, Medline, Google Scholar