Brain Structure Abnormalities in Early-Onset and Adolescent-Onset Conduct Disorder
Abstract
Objective:
The developmental taxonomic theory proposes that neurodevelopmental factors play a critical role in the etiology of early-onset conduct disorder, whereas adolescent-onset conduct disorder arises as a result of social mimicry of deviant peers. Recent studies have challenged this theory by demonstrating that adolescents with both early- and adolescent-onset forms of conduct disorder show impaired emotional learning and abnormal neural activation during facial expression processing. The present study extends this work by investigating brain structure in both subtypes of conduct disorder.
Method:
Voxel-based morphometry was used to compare gray matter volumes in four regions of interest (amygdala, insula, anterior cingulate, and orbitofrontal cortex) in male adolescents with early-onset (N=36) or adolescent-onset (N=27) conduct disorder and in healthy comparison subjects (N=27). Whole-brain structural analyses were also performed.
Results:
The combined conduct disorder group displayed gray matter volume reductions in the bilateral amygdala, extending into the insula, relative to healthy comparison subjects. Separate comparisons between healthy subjects and each conduct disorder subgroup revealed lower amygdala volume in both subgroups and reduced right insula volume in the adolescent-onset subgroup. Regression analyses within the conduct disorder subjects alone demonstrated a negative correlation between conduct disorder symptoms and right insula volume.
Conclusions:
The results demonstrate that gray matter volume reductions in brain regions involved in processing socioemotional stimuli are associated with conduct disorder, regardless of age of onset. Brain structural abnormalities may contribute to the emergence of adolescent-onset as well as early-onset conduct disorder.
Conduct disorder is a psychiatric condition that emerges in either childhood or adolescence and is characterized by increased aggressive and antisocial behavior (1). The societal effect of conduct disorder is profound because individuals with this disorder are often impaired across multiple settings (e.g., social, educational, and interpersonal) and are at increased risk for developing mental and physical health problems in adulthood (2, 3).
The role of neurobiological factors in the etiology of conduct disorder and related disorders has attracted increased attention in recent years (4–6). However, a significant obstacle to our progress in characterizing the pathophysiology of conduct disorder is the heterogeneity of the phenotype. In her influential developmental taxonomic theory, Terrie E. Moffitt, Ph.D. (7), along with her colleagues (8), argued that early-onset conduct disorder is a neurodevelopmental condition, whereas adolescent-onset conduct disorder emerges as a result of social mimicry and therefore does not have a neurological basis. As a consequence, all previous structural neuroimaging studies of conduct disorder (9–11) have focused exclusively on the early-onsetsubtype. However, prior research has demonstrated similar impairments in fear conditioning (12), affective decision making (13), and facial expression recognition (14) in both conduct disorder subtypes. A recent neuroimaging study also found abnormal activation in brain regions involved in affective behavior, including the amygdala, during facial expression processing in both early- and adolescent-onset conduct disorder (15). Our primary aim in the present study was to examine whether these behavioral and functional neural abnormalities are underpinned by similar changes in brain structure in the two conduct disorder subtypes. On the basis of previous research, we predicted that structural abnormalities in brain regions involved in affective behavior would be observed in both conduct disorder subtypes relative to comparison subjects.
A number of studies have reported increased levels of psychopathic or callous-unemotional traits in children and adolescents with conduct problems or conduct disorder diagnoses (13, 16, 17). However, previous structural neuroimaging studies of conduct disorder (10, 11) have not taken psychopathic or callous-unemotional traits into account. In contrast, the only study to have investigated brain structural correlates of conduct problems and callous-unemotional traits, conducted by de Brito et al. (18), did not employ DSM-IV criteria for conduct disorder. The authors observed increased gray matter concentration in the anterior cingulate and medial orbitofrontal cortex in children with conduct problems and callous-unemotional traits, whereas previous studies of conduct disorder (10, 11) found reduced gray matter volume in the amygdala, anterior insula, and orbitofrontal cortex. This led de Brito et al. (18) to propose that conduct disorder with callous-unemotional traits is associated with a qualitatively different neurological profile compared with conduct disorder without callous-unemotional traits. Unfortunately, the study did not include a group with conduct problems and low levels of callous-unemotional traits, which would have provided a stronger test of this hypothesis. To further evaluate this proposal, our second aim was to investigate the consequences of individual variation in psychopathic or callous-unemotional traits on brain structure. Given previous functional neuroimaging evidence (15) that increased conduct disorder symptoms are associated with greater brain abnormalities, regardless of the conduct disorder subtype or level of callous-unemotional traits, our third aim was to investigate relationships between conduct disorder symptoms and structural abnormalities.
The present study also incorporated a methodological advance in relation to previous structural imaging studies of conduct disorder by employing the recently developed Diffeomorphic Anatomical Registration Through Exponentiated Lie algebra image registration procedure (19). This algorithm improves realignment of small inner structures (20), enhancing image registration across participants and allowing better detection of volumetric differences in subcortical structures (21).
Method
Participants
Sixty-five male adolescents with conduct disorder were re-cruited from schools, pupil referral units, and the Cambridge Youth Offending Service, Cambridge, United Kingdom. All participants gave written informed consent to participate in the study, which was approved by the local research ethics committee. Exclusion criteria for the conduct disorder group included an IQ <80, as estimated using the Wechsler Abbreviated Scale of Intelligence (22), or presence of a pervasive developmental disorder (e.g., autism). A healthy comparison group (no history of conduct disorder/oppositional defiant disorder and no current psychiatric illness) of 27 male adolescents, matched for IQ, was recruited from schools and colleges. The majority (N=75) of healthy comparison subjects and conduct disorder participants had participated in our earlier functional magnetic resonance imaging (MRI) study (15).
All participants were assessed for conduct disorder, oppositional defiant disorder, attention deficit hyperactivity disorder (ADHD), major depressive disorder, generalized anxiety disorder, obsessive-compulsive disorder, posttraumatic stress disorder, and substance dependence using the Schedule for Affective Disorders and Schizophrenia for School-Age Children–Present and Lifetime Version (23). Diagnostic interviews were carried out separately with participants and caregivers. Participants were diagnosed with early-onset conduct disorder if they or their caregivers reported that at least one conduct disorder symptom and functional impairment was present prior to age 10 years (1). If symptom onset occurred after age 10 years, a diagnosis of adolescent-onset conduct disorder was given. According to these criteria, 37 participants were classified as having early-onset conduct disorder and 28 as having the adolescent-onset subtype.
Callous-unemotional and overall psychopathic traits were assessed in all participants using the Callous-Unemotional Dimension subscale and the total score of the self-report Youth Psychopathic traits Inventory (24), respectively. Data on callous-unemotional traits were also obtained from parents using the Inventory of Callous-Unemotional traits (25). Because of time constraints or parents electing not to complete the questionnaire, data from this inventory were only available for 73 participants. Anxiety was assessed using the Spielberger State-Trait Anxiety Inventory (26). The Adolescent Alcohol and Drug Involvement Scale (27) measured alcohol and substance use. Finally, socioeconomic status was quantified using the ACORN geodemographic tool (http://www.caci.co.uk/acorn-classification.aspx).
Neuroimaging
Data acquisition.
Structural MRI was acquired using a 3-Tesla Siemens Tim Trio at the Medical Research Council Cognition and Brain Sciences Unit (Cambridge, United Kingdom). We acquired T1-weighted three-dimensional magnetization-prepared rapid gradient-echoimages (voxel size=1×1×1 mm, repetition time=2,250 msec, echo time=2.99 msec, inversion time=900 msec, flip angle=9°). Total scanning time was 4 minutes and 16 seconds.
Image processing and analysis.
Voxel-based morphometry analysis was performed using SPM5 (Wellcome Trust Department of Imaging Neuroscience, London). Images were first inspected for scanner artifacts and gross neuroanatomical abnormalities, such as tumors or cysts, by a consultant neurologist and a consultant radiologist (two conduct disorder participants were excluded for this reason [early-onset, N=1; adolescent-onset, N=1]). We then employed the Diffeomorphic Anatomical Registration Through Exponentiated Lie algebra toolbox in SPM5 to spatially segment the images; import the images into native space; create a template from the merged images of the 90 subjects; and warp, modulate, normalize, and smooth the individual results using an 8-mm full-width at half-maximum Gaussian kernel.
Following preprocessing, statistical analyses were performed in SPM5 using a general linear model to permit quantification of group effects (F statistics and follow-up t tests), with total gray matter volume as a covariate of no interest. We first performed a region-of-interest analysis using a significance level of p<0.05, with family-wise error correction for multiple comparisons within the regions of interest (i.e., small-volume correction). In line with previous imaging studies on antisocial populations (10, 11, 15, 28), we defined the amygdala, insula, anterior cingulate, and orbitofrontal cortex as our a priori regions of interest using the atlas for Automated Anatomical Labeling (29). The number of voxels within each region of interest was as follows: amygdala: 248; insula: 1,858; anterior cingulate: 1,399; orbitofrontal cortex: 3,645. We subsequently performed comparisons between groups on the whole-brain level (employing a statistical threshold of p≤0.001 uncorrected, ≥10 contiguous voxels). Lastly, we used regression analyses to examine whether self-reported overall psychopathic or callous-unemotional traits, parent-reported callous-unemotional traits, or conduct disorder symptoms (lifetime/ever, current, or aggressive symptoms) were related to gray matter volume when considering the conduct disorder participants alone (N=63).
Results
Demographic and Clinical Variables
The study groups were matched on estimated full-scale IQ, ethnicity, handedness, and state and trait anxiety, but early-onset conduct disorder participants were slightly younger (p<0.05) and of lower socioeconomic status (exact test: p=0.005) relative to healthy comparison subjects (Table 1).
Group | ||||||||
---|---|---|---|---|---|---|---|---|
Measure | 1) Healthy Comparison (N=27) | 2) Adolescent-Onset Conduct Disorder (N=27) | 3) Early-Onset Conduct Disorder (N=36) | Analysis | ||||
Mean | SD | Mean | SD | Mean | SD | p | Post Hoc | |
Age (years)a | 18.53 | 1.04 | 17.89 | 1.09 | 17.69 | 1.16 | 0.01 | 1>3 |
Estimated full-scale IQ | 101.41 | 9.05 | 100.89 | 9.56 | 97.72 | 8.11 | 0.20 | |
Psychopathic traits (Youth Psychopathic traits Inventory total score) | 1.98 | 0.28 | 2.47 | 0.34 | 2.46 | 0.42 | <0.001 | 1< (2, 3) |
Youth Psychopathic traits Inventory callous-unemotional subscale score | 0.61 | 0.10 | 0.74 | 0.11 | 0.74 | 0.12 | <0.001 | 1< (2, 3) |
Inventory of Callous-Unemotional traits score | 21.44 | 7.63 | 31.78 | 11.17 | 36.62 | 15.03 | <0.001 | 1< (2, 3) |
Lifetime conduct disorder symptoms | 0.41 | 0.64 | 7.48 | 2.46 | 9.11 | 1.85 | <0.001 | 1< 2 < 3 |
Current conduct disorder symptoms | 0.07 | 0.27 | 4.78 | 1.80 | 4.97 | 2.56 | <0.001 | 1< (2, 3) |
Aggressive conduct disorder symptoms | 0.07 | 0.27 | 2.93 | 1.38 | 3.56 | 1.03 | <0.001 | 1< 2 < 3 |
Lifetime ADHD symptoms | 2.62 | 2.38 | 5.96 | 4.27 | 8.74 | 4.86 | <0.001 | 1< 2 < 3 |
Current ADHD symptoms | 1.42 | 1.94 | 3.92 | 3.71 | 6.41 | 4.92 | <0.001 | (1, 2) <3 |
State Anxiety (Spielberger Trait Anxiety Inventory score) | 31.78 | 6.61 | 29.93 | 7.26 | 28.83 | 6.16 | 0.22 | |
Trait Anxiety (Spielberger Trait Anxiety Inventory score) | 36.48 | 8.72 | 37.85 | 8.38 | 39.65 | 8.91 | 0.36 | |
N | % | N | % | N | % | p | Post Hoc | |
ACORN socioeconomic status | 0.02 | 1>3 | ||||||
Wealthy achievers | 5 | 18.5 | 2 | 7.4 | 0 | 0 | ||
Urban prosperity | 7 | 25.9 | 6 | 22.2 | 2 | 5.6 | ||
Comfortably off | 6 | 22.2 | 6 | 22.2 | 16 | 44.4 | ||
Moderate means | 2 | 7.4 | 1 | 3.7 | 4 | 11.1 | ||
Hard-pressed | 7 | 25.9 | 12 | 44.4 | 14 | 38.9 | ||
Ethnicity | 0.66 | |||||||
Caucasian | 26 | 96.3 | 25 | 92.6 | 35 | 97.2 | ||
Non-Caucasian | 1 | 3.7 | 2 | 7.4 | 1 | 2.8 |
TABLE 1. Characteristics of Male Adolescents With Early- or Adolescent-Onset Conduct Disorder and Healthy Comparison Subjects in the Structural Imaging Analysesa
As expected, the study groups differed in current, lifetime/ever, and aggressive conduct disorder symptoms (F=60.49, df=2, 87, p<0.001; F=189.91, df=2, 87, p<0.001; F=98.72, df=2, 87, p<0.001, respectively), with both conduct disorder subgroups reporting higher numbers of symptoms than comparison subjects on all three measures (p<0.001). Early-onset conduct disorder participants endorsed more lifetime/ever conduct disorder symptoms (p<0.005) and more aggressive symptoms (p<0.05) than adolescent-onset participants, but the two subgroups did not differ in current conduct disorder symptoms. The study groups also differed in current and lifetime/ever ADHD symptoms (F=12.36, df=2, 82, p<0.001; F=16.65, df=2, 82, p<0.001, respectively), with early-onset participants reporting higher levels of current ADHD symptoms than adolescent-onset participants (p<0.05) and comparison subjects (p<0.001). Early-onset participants also reported higher levels of lifetime/ever ADHD symptoms than adolescent-onset participants (p<0.05) and comparison subjects (p<0.001), and the adolescent-onset subgroup had higher levels of lifetime/ever symptoms than comparison subjects (p<0.05).
There were main effects of group on self-reported overall psychopathic (F=17.13, df=2, 87, p<0.001) and callous-unemotional (F=11.81, df=2, 87, p<0.001) traits, with adolescent- and early-onset conduct disorder participants scoring higher than comparison subjects on both measures (p<0.001). The conduct disorder subgroups did not differ from each other on either measure. Finally, the study groups differed on parent-reported callous-unemotional traits (F=11.03, df=2, 71, p<0.001), with both conduct disorder subgroups having higher scores than comparison subjects (both p<0.005) but not differing from each other.
Neuroimaging
Group effects on gray matter volume.
There were no group differences in total gray matter volume. When the three groups were compared using region-of-interest analysis, a main effect of group was observed in the bilateral amygdala (left: p=0.04, family-wise error [small-volume correction]; right: p=0.02, family-wise error [small-volume correction]). Further group effects outside the regions of interest are listed in Table 1 of the data supplement accompanying the online version of this article.
Comparisons between the combined conduct disorder group and healthy comparison subjects.
To decompose the group effects, we first compared the combined conduct disorder group (early-onset plus adolescent-onset) with the healthy comparison group. Relative to comparison subjects, the combined conduct disorder group showed reduced gray matter volume in the bilateral amygdala (Table 2, Figure 1), extending into the ventral insula on the left side. Other brain areas outside of the regions of interest that were reduced in volume in conduct disorder participants are listed in Table 2. These included the left dorsomedial prefrontal cortex and bilateral caudate nucleus. None of the regions of interest showed increased volume in the combined conduct disorder group relative to comparison subjects. However, the combined group displayed increased volume in the left frontal operculum and left inferior temporal gyrus (both p values <0.001, uncorrected).
Cerebral Region and Hemisphere | Local Maxima (Z) | Number of Significant Voxels in Cluster | Montreal Neurological Institute Coordinates (x, y, z) |
---|---|---|---|
Amygdala | |||
Left | 3.55* | 167 | –30, 5, –18 |
Right | 3.68* | 348 | 26, 3, –17 |
Insula: left | 3.33 | 50 | –32, 9, –18 |
Dorsomedial prefrontal cortex: left | 3.61 | 211 | –6, 66, 25 |
Caudate nucleus | |||
Left | 3.68 | 113 | –15, –18, 19 |
Right | 3.57 | 91 | 16, –18, 22 |
Fusiform gyrus: left | 3.64 | 48 | –38, –79, –14 |
Inferior occipital cortex: left | 4.26 | 193 | –24, –88, –5 |
Superior occipital cortex: left | 3.40 | 129 | –18, –82, 27 |
TABLE 2. Brain Regions Reduced in Gray Matter Volume in the Combined Conduct Disorder Group (Early-Onset Plus Adolescent-Onset) Relative to Comparison Subjectsa
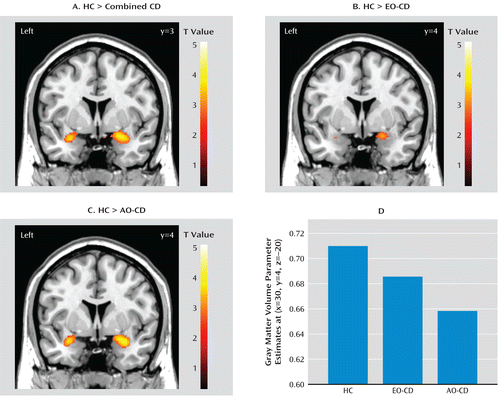
FIGURE 1. Statistical Parametric Mapping Results in Male Adolescents With Early- or Adolescent-Onset Conduct Disorder and Healthy Comparison Subjectsa
a The images depict A) foci of gray matter volume reductions in the bilateral amygdala (left: x=–30, y=5, z=–18; right: x=26, y=3, z=–17) in a combined group of participants with conduct disorder (N=63) relative to healthy comparison subjects (N=27); B) significant bilateral amygdala (left: x=–30, y=5, z=–18; right: x=24, y=4, z=–17) volume reduction in participants with early-onset conduct disorder relative to healthy comparison subjects; C) significant bilateral amygdala (left: x=–30, y=5, z=–18; right: x=30, y=4, z=–20) volume reduction in participants with adolescent-onset conduct disorder relative to healthy comparison subjects; and D) the mean value for right amygdala gray matter volume in each group. The color bars represent T statistics. (For cluster sizes and Z scores, see Table 2 and Table 3.) Images are thresholded at p<0.005, uncorrected, for display purposes. Abbreviations: HC=healthy comparison; CD=conduct disorder; EO=early-onset; AO=adolescent-onset.
Comparisons between each conduct disorder subgroup and healthy comparison subjects.
Relative to comparison subjects, both early- and adolescent-onset conduct disorder participants showed reduced right amygdala gray matter volume. Adolescent-onset participants also displayed reduced left amygdala volume, and reduced left amygdala volume in early-onset participants nearly reached statistical significance (p=0.06, family-wise error [small-volume correction] [Figure 1, Table 3]).
Cerebral Region and Hemisphere | Local Maxima (Z) | Number of Significant Voxels in Cluster | Montreal Neurological Institute Coordinates (x, y, z) |
---|---|---|---|
Healthy comparison>early-onset conduct disorder | |||
Amygdala | |||
Left | 2.78b | 125 | –30, 5, –18 |
Right | 2.96* | 235 | 24, 4, –17 |
Caudate nucleus | |||
Left | 3.77 | 106 | –15, –18, 22 |
Right | 3.55 | 87 | 16, –18, 22 |
Fusiform gyrus: left | 3.59 | 50 | –36, –79, –14 |
Inferior occipital cortex: left | 3.97 | 935 | –24, –88, –5 |
Cerebellum | |||
Left | 3.12 | 38 | –12, –43, –26 |
Right | 3.52 | 196 | 24, –52, –32 |
Early-onset conduct disorder>healthy comparison | |||
Inferior frontal gyrus: left | 3.43 | 207 | –54, 29, 16 |
Middle temporal gyrus: left | 3.09 | 35 | –46, 4, –33 |
Supplementary motor area | |||
Left | 3.57 | 496 | –9, 15, 63 |
Right | 3.26 | 189 | 6, –10, 66 |
Healthy comparison>adolescent-onset conduct disorder | |||
Amygdala | |||
Left | 3.50* | 123 | –30, 5, –18 |
Right | 3.93* | 382 | 30, 4, –20 |
Insula: right | 3.54* | 17 | 32, 9, –18 |
Orbitofrontal cortex: left | 3.23 | 27 | –16, 50, –23 |
Dorsomedial prefrontal cortex: left | 3.84 | 335 | –5, 65, 29 |
Inferior occipital cortex: left | 3.87 | 218 | –24, –87, –3 |
Mid-occipital cortex: right | 3.71 | 185 | 40, –82, 4 |
Adolescent-onset conduct disorder>healthy comparison | |||
Frontal operculum | |||
Left | 4.03 | 521 | –51, 12, 22 |
Right | 3.63 | 190 | 54, 9, 4 |
Inferior temporal gyrus: left | 3.20 | 70 | –46, –3, –35 |
Superior temporal gyrus: left | 3.16 | 77 | –39, 11, –35 |
Middle temporal gyrus: right | 3.17 | 79 | 48, 8, –30 |
TABLE 3. Separate Comparisons Between Each Conduct Disorder Subgroup and Healthy Comparison Subjects a
None of the other regions of interest showed significant reductions in gray matter volume in early-onset participants relative to comparison subjects (for additional regions that were decreased in volume in early-onset participants, see Table 3).
As well as reduced bilateral amygdala volume, adolescent-onset participants showed reduced gray matter volume in the right ventral insula, left orbitofrontal cortex, and left dorsomedial prefrontal cortex. Coordinates of these differences and additional regions outside of the regions of interest that were reduced in volume in adolescent-onset participants are listed in Table 3. None of the regions of interest were increased in volume in adolescent- or early-onset participants relative to comparison subjects.
Comparisons between the conduct disorder subgroups.
A direct comparison of the conduct disorder subgroups revealed no significant differences in the regions of interest. However, gray matter volume differences between the subgroups were detected outside the regions of interest (see Table 2 in the data supplement).
Potential Confounds
To examine whether group differences in demographic, clinical, and personality variables contributed to the aforementioned findings, we reran our voxel-based morphometry analyses, adjusting for these variables in separate general linear model analyses. Relative to comparison subjects, the combined conduct disorder group continued to show reduced bilateral amygdala volume (p<0.05, family-wise error [small-volume correction]) when adjusting for ADHD symptoms, age, socioeconomic status, tobacco, alcohol, or cannabis use, self-reported psychopathic or callous-unemotional traits, and parent-reported callous-unemotional traits (in a subset of the sample, N=73). The effect in the left insula was still significant (p<0.05) when controlling for each of these variables, with the exception of ADHD symptoms or age.
Relative to comparison subjects, adolescent-onset participants continued to show significant reduced bilateral amygdala volume (p<0.05, family-wise error [small-volume correction]) when controlling for each of these variables. They also displayed lower right insula volume when adjusting for socioeconomic status, self-reported psychopathic or callous-unemotional traits, parent-reported callous-unemotional traits, and alcohol or cannabis use, although this difference fell short of statistical significance (p≤0.10, family-wise error [small-volume correction]) when controlling for ADHD symptoms, age, or tobacco use.
When adjusting for ADHD symptoms, socioeconomic status, alcohol or cannabis use, and self-reported psychopathic or callous-unemotional traits, early-onset participants continued to show significant reduced volume or reduced volume that nearly reached statistical significance in the right amygdala (all p values ≤0.06, family-wise error [small-volume correction]). However, this finding was diminished to only marginal significance when adjusting for age (p=0.08, family-wise error [small-volume correction]) and tobacco use (p=0.09, family-wise error [small-volume correction]) and was abolished when we controlled for parent-reported callous-unemotional traits. The aforementioned findings in the left amygdala remained intact when we controlled for socioeconomic status, self-reported psychopathic or callous-unemotional traits, and alcohol use (all p values ≤0.06, family-wise error [small-volume correction]), but findings were no longer even marginally significant after adjustment for ADHD symptoms, parent-reported callous-unemotional traits, and tobacco or cannabis use.
Correlations Between Conduct Disorder Symptoms and Gray Matter Volume in Conduct Disorder Participants Only
Right insula volume was negatively correlated with lifetime/ever conduct disorder symptoms (Z=3.57, p<0.05, family-wise error [small-volume correction] [Figure 2]). This negative correlation was still significant (p<0.05, family-wise error [small-volume correction]) when adjusting for self-reported callous-unemotional traits but fell short of significance (p=0.06, family-wise error [small-volume correction]) when adjusting for self-reported psychopathic traits. There was also a negative correlation (Z=3.09, p=0.001, uncorrected) when controlling for lifetime/ever ADHD symptoms. However, in the subset of conduct disorder participants for whom Inventory of Callous-Unemotional traits data were available (N=47), the negative correlation in the right insula was no longer significant when we controlled for parent-reported callous-unemotional traits. None of the other regions of interest showed a significant positive or negative correlation with lifetime/ever or current conduct disorder symptoms.
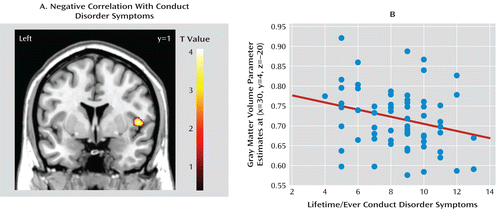
FIGURE 2. Negative Correlation Between Right Insula Gray Matter Volume and Lifetime/Ever Conduct Disorder Symptoms in Conduct Disorder Participants Onlya
a The image A) depicts the negative correlation in the right insula (x=47, y=2, z=4). The color bar represents T statistics. The image is thresholded at p<0.005, uncorrected, for display purposes. The image shown in B is a scatter plot depicting the negative correlation between gray matter volume and lifetime/ever conduct disorder symptoms at the peak voxel in the right insula (as shown in A).
Additional regions outside of the regions of interest that were positively or negatively correlated with lifetime/ever conduct disorder symptoms are listed in Table 3 of the data supplement.
Regression Analyses Using Callous-Unemotional and Psychopathic Traits in Conduct Disorder Participants Only
We did not observe any negative or positive correlations between self-reported psychopathic or callous-unemotional traits and volumes in the regions of interest. However, there was a positive correlation between self-reported callous-unemotional traits and volume in the caudate nucleus (Z=3.94, p<0.001, uncorrected) and ventral striatum (Z=3.21, p<0.001, uncorrected [see Figure 1 in the data supplement]). This correlation remained significant (p<0.001, uncorrected) when adjusting for conduct disorder symptoms, suggesting that self-reported callous-unemotional traits are associated with striatal volume, independent of the behavioral features of conduct disorder. Although weaker, there was also a positive correlation between self-reported overall psychopathic traits and right caudate volume (Z=3.02, p=0.001, uncorrected).
In the subset of conduct disorder participants for whom parent-reported callous-unemotional trait data were available (N=47), no region of interest was negatively or positively correlated with parent-reported callous-unemotional traits. There was also no positive relationship between striatal volume and parent-reported callous-unemotional traits.
Discussion
Our results for the early-onset conduct disorder subgroup are broadly consistent with previous findings showing reduced amygdala gray matter volume (10, 11) and reduced temporal lobe volume (9) in male children and adolescents with early-onset conduct disorder. A key novel finding of this study is that reduced gray matter volume in limbic regions putatively involved in the pathophysiology of conduct disorder (e.g., the amygdala) is also observed in the adolescent-onset subtype. Reduced amygdala volume therefore appears to be a general characteristic of male adolescents with conduct disorder, irrespective of age of onset. This finding did not appear to be explained by increased levels of ADHD symptoms, psychopathic traits, or callous-unemotional traits in the conduct disorder participants, since it remained significant when adjusting for these variables. We also demonstrated a negative correlation between lifetime/ever conduct disorder symptoms and right insula volume, indicating that increased severity of conduct disorder is associated with greater structural abnormalities in brain regions involved in affective behavior. In addition to the amygdala and insula, we observed reduced gray matter volume in the dorsomedial prefrontal cortex and caudate nucleus in participants with conduct disorder. Finally, there were no volumetric differences between early- and adolescent-onset conduct disorder participants in our a priori regions of interest.
Contrary to the developmental taxonomic theory (7, 8), we demonstrated that participants with both subtypes of conduct disorder show similar reductions in gray matter volume in brain regions, including the amygdala. Surprisingly, the reductions in amygdala volume were numerically greater and statistically more robust in the adolescent-onset subgroup than the early-onset subgroup. These reductions in amygdala volume may have underpinned our earlier behavioral and functional neuroimaging findings, since this structure plays a critical role in fear conditioning (30, 31) and is activated in functional MRI (fMRI) paradigms involving facial expression processing (32). Structural abnormalities in the amygdala may also underlie the finding that both early- and adolescent-onset conduct disorder are associated with blunted cortisol responses to stress (33), since the amygdala is involved in initiating hypothalamic-pituitary-adrenal axis responses to stress (34).
Consistent with earlier work (11), we observed reduced insula volume in participants with conduct disorder, although the insula regions were located more ventrally than those reported previously. Interestingly, we also observed a negative correlation between conduct disorder symptoms and right insula volume when considering conduct disorder participants only. The insula is implicated in many aspects of affective behavior, including awareness of one's own emotional states (35) and those of others (36). Structural changes in the insula may therefore contribute to the empathy deficits observed in children and adolescents with conduct disorder (11, 37, 38). The insula is also activated in tasks involving the processing of negative cues and is implicated in the pathophysiology of anxiety (39). This may explain why individuals with conduct disorder show reduced autonomic responses to punishing or aversive stimuli (40, 41) and fail to take potential losses into account when making decisions (12). Although we did not replicate the finding of reduced left orbitofrontal cortex volume in early-onset conduct disorder (10), reduced left orbitofrontal cortex volume was observed in adolescent-onset conduct disorder participants relative to comparison subjects. We also observed reduced dorsomedial prefrontal cortex volume in the adolescent-onset subgroup and the combined conduct disorder group relative to comparison subjects. The dorsomedial prefrontal cortex is implicated in mentalizing, episodic memory retrieval, and executive control (42). Of interest, conduct disorder participants showed reduced activation of the dorsomedial prefrontal cortex during face processing in our previous functional neuroimaging study (15). These structural findings therefore provide preliminary evidence for deficits in prefrontal cortex regions involved in mentalizing and executive control in conduct disorder.
In relation to the second aim of the study, no negative or positive correlations were observed between self-reported psychopathic or callous-unemotional traits or parent-reported callous-unemotional traits and gray matter volume in the regions of interest. The lack of a negative correlation between callous-unemotional traits and amygdala volume may be considered surprising given earlier reports of reduced amygdala responses to fearful faces in children with conduct problems and callous-unemotional traits (43, 44) but is consistent with the structural neuroimaging literature, which has failed to demonstrate a negative relationship between psychopathic or callous-unemotional traits and amygdala volume using voxel-based morphometry or manual tracing methods (18, 45–47). We were also unable to replicate the findings of de Brito et al. (18), since neither self-reported nor parent-reported callous-unemotional traits were positively correlated with medial orbitofrontal cortex or anterior cingulate volume. Interestingly, we observed a positive correlation between self-reported callous-unemotional traits and caudate nucleus and ventral striatal volumes, which is consistent with a recent study that reported increased striatal volume in adults with psychopathy (48). Increased volume in structures involved in reward processing may also underlie fMRI results showing enhanced ventral striatal activity during anticipation of monetary rewards in healthy volunteers who score high in psychopathic traits (49).
Limitations
First, measuring age of conduct disorder onset using retrospective reports was not optimal. To counter this problem, we obtained extremely detailed data from standardized diagnostic interviews with parents as well as participants, and we used a number of strategies to enhance accurate recall, such as asking informants to consider important life landmarks when recalling the onset of symptoms. Future studies investigating similar issues may wish to utilize participants from birth cohort or longitudinal studies for whom externalizing data from childhood and adolescence are available. Second, in common with the vast majority of neuroimaging studies of psychiatric disorders, our findings are cross-sectional, meaning we cannot infer that the structural abnormalities observed in individuals with early- or adolescent-onset conduct disorder have played a causal role in the etiology of their antisocial or violent behavior. While we note that our results are broadly consistent with developmental theories that link amygdala dysfunction with antisocial behavior via impairments in reinforcement learning (4) and sensitivity to distress cues (50), prospective studies are needed to assess whether amygdala deficits are a cause or a consequence of conduct disorder. Finally, since all participants were male adolescents, our findings may not generalize to female adolescents.
Conclusions
This is the largest structural neuroimaging study to date to investigate the neural basis of conduct disorder and the first to investigate the developmental taxonomic theory of antisocial behavior. We observed amygdala gray matter volume reductions in both early- and adolescent-onset conduct disorder that could not be accounted for by comorbid ADHD symptoms, self-reported psychopathic or callous-unemotional traits, age, socioeconomic status, IQ, or substance abuse. Furthermore, there were no significant volumetric differences between the conduct disorder subgroups in the regions of interest. Our results therefore support the proposal that both forms of conduct disorder may stem from dysfunction in neural circuits involved in emotion processing, contrary to the developmental taxonomic theory. We also observed a negative correlation between insula volume and lifetime/ever conduct disorder symptoms when considering the conduct disorder participants alone, indicating that increased severity of the disorder is associated with greater structural abnormalities in brain regions involved in processing socioemotional stimuli and providing support for dimensional approaches to understanding disruptive behavior disorders.
1.
2. : Prediction of differential adult health burden by conduct problem subtypes in males. Arch Gen Psychiatry 2007; 64:476–484Crossref, Medline, Google Scholar
3. : Show me the child at seven: the consequences of conduct problems in childhood for psychosocial functioning in adulthood. J Child Psychol Psychiatry 2005; 46:837–849Crossref, Medline, Google Scholar
4. : The development of psychopathy. J Child Psychol Psychiatry 2006; 47:262–276Crossref, Medline, Google Scholar
5. : The evidence for a neurobiological model of childhood antisocial behavior. Psychol Bull 2007; 133:149–182Crossref, Medline, Google Scholar
6. Hodgins SViding EPlodowski A (eds): The Neurobiological Basis of Violence: Science and Rehabilitation. Oxford, United Kingdom, Oxford University Press, 2009Google Scholar
7. : Adolescence-limited and life-course-persistent antisocial behavior: a developmental taxonomy. Psychol Rev 1993; 100:674–701Crossref, Medline, Google Scholar
8. : Research review: DSM-V conduct disorder: research needs for an evidence base. J Child Psychol Psychiatry 2008; 49:3–33Crossref, Medline, Google Scholar
9. : Reduced temporal lobe volume in early onset conduct disorder. Psychiatry Res 2004; 132:1–11Crossref, Medline, Google Scholar
10. : Morphometric brain abnormalities in boys with conduct disorder. J Am Acad Child Adolesc Psychiatry 2008; 47:540–547Crossref, Medline, Google Scholar
11. : A structural neural deficit in adolescents with conduct disorder and its association with lack of empathy. Neuroimage 2007; 37:335–342Crossref, Medline, Google Scholar
12. : Fear conditioning and affective modulation of the startle reflex in male adolescents with early-onset or adolescence-onset conduct disorder and healthy control subjects. Biol Psychiatry 2008; 63:279–285Crossref, Medline, Google Scholar
13. : Decision making and executive function in male adolescents with early-onset or adolescence-onset conduct disorder and control subjects. Biol Psychiatry 2009; 66:162–168Crossref, Medline, Google Scholar
14. : Deficits in facial expression recognition in male adolescents with early-onset or adolescence-onset conduct disorder. J Child Psychol Psychiatry 2009; 50:627–636Crossref, Medline, Google Scholar
15. : Neural abnormalities in early-onset and adolescence-onset conduct disorder. Arch Gen Psychiatry 2010; 67:729–738Crossref, Medline, Google Scholar
16. : The association of psychopathic traits with aggression and delinquency in non-referred boys and girls. Behav Sci Law 2005; 23:803–817Crossref, Medline, Google Scholar
17. : Psychopathic traits and conduct problems in community and clinic-referred samples of children: further development of the psychopathy screening device. Psychol Assess 2000; 12:382–393Crossref, Medline, Google Scholar
18. : Size matters: increased grey matter in boys with conduct problems and callous-unemotional traits. Brain 2009; 132:843–852Crossref, Medline, Google Scholar
19. : A fast diffeomorphic image registration algorithm. Neuroimage 2007; 38:95–113Crossref, Medline, Google Scholar
20. : A quantitative evaluation of cross-participant registration techniques for MRI studies of the medial temporal lobe. Neuroimage 2009; 44:319–327Crossref, Medline, Google Scholar
21. : Can voxel based morphometry, manual segmentation and automated segmentation equally detect hippocampal volume differences in acute depression? Neuroimage 2009; 45:29–37Crossref, Medline, Google Scholar
22. : Wechsler Abbreviated Scale of Intelligence (WASI). San Antonio, Tex, Psychological Corporation, 1999Google Scholar
23. : Schedule for Affective Disorders and Schizophrenia for School-Age Children–Present and Lifetime Version (K-SADS-PL): initial reliability and validity data. J Am Acad Child Adolesc Psychiatry 1997; 36:980–988Crossref, Medline, Google Scholar
24. : Psychopathic traits in non-referred youths: a new assessment tool, in Psychopaths: New International Perspectives. Edited by Blaauw ESheridan L. the Hague, the Netherlands, Elsevier, 2002Google Scholar
25. : Callous-unemotional traits in a community sample of adolescents. Assessment 2006; 13:454–469Crossref, Medline, Google Scholar
26. : Manual for the State-Trait Anxiety Inventory. Palo Alto, Calif, Consulting Psychologists Press, 1983Google Scholar
27. : The Adolescent Alcohol and Drug Involvement Scale. Madison, Wisc, Center for Health Policy and Program Evaluation, 2000Google Scholar
28. : Sex differences in orbitofrontal gray as a partial explanation for sex differences in antisocial personality. Mol Psychiatry 2011; 16:227–236Crossref, Medline, Google Scholar
29. : Automated anatomical labeling of activations in SPM using a macroscopic anatomical parcellation of the MNI MRI single-subject brain. Neuroimage 2002; 15:273–289Crossref, Medline, Google Scholar
30. : Impaired fear conditioning following unilateral temporal lobectomy in humans. J Neurosci 1995; 15:6846–6855Crossref, Medline, Google Scholar
31. : Brain systems mediating aversive conditioning: an event-related fMRI study. Neuron 1998; 20:947–957Crossref, Medline, Google Scholar
32. : A differential neural response in the human amygdala to fearful and happy facial expressions. Nature 1996; 383:812–815Crossref, Medline, Google Scholar
33. : Cortisol diurnal rhythm and stress reactivity in male adolescents with early-onset or adolescence-onset conduct disorder. Biol Psychiatry 2008; 64:599–606Crossref, Medline, Google Scholar
34. : The neurobiology of stress and development. Annu Rev Psychol 2007; 58:145–173Crossref, Medline, Google Scholar
35. : How do you feel—now? The anterior insula and human awareness. Nat Rev Neurosci 2009; 10:59–70Crossref, Medline, Google Scholar
36. : Empathy for pain involves the affective but not sensory components of pain. Science 2004; 303:1157–1162Crossref, Medline, Google Scholar
37. : Empathy in conduct-disordered and comparison youth. Dev Psychol 1996; 32:988–998Crossref, Google Scholar
38. : Empathy in boys with disruptive behavior disorders. J Child Psychol Psychiatry 2005; 46:867–880Crossref, Medline, Google Scholar
39. : An insular view of anxiety. Biol Psychiatry 2006; 60:383–387Crossref, Medline, Google Scholar
40. : Facial expression recognition, fear conditioning and startle modulation in female subjects with conduct disorder. Biol Psychiatry 2010; 68:272–279Crossref, Medline, Google Scholar
41. : Impaired decision making in oppositional defiant disorder related to altered psychophysiological responses to reinforcement. Biol Psychiatry 2010; 68:337–344Crossref, Medline, Google Scholar
42. : Functional specialization within rostral prefrontal cortex (area 10): a meta-analysis. J Cogn Neurosci 2006; 18:932–948Crossref, Medline, Google Scholar
43. : Reduced amygdala response to fearful expressions in children and adolescents with callous-unemotional traits and disruptive behavior disorders. Am J Psychiatry 2008; 165:712–720Link, Google Scholar
44. : Amygdala hypoactivity to fearful faces in boys with conduct problems and callous-unemotional traits. Am J Psychiatry 2009; 166:95–102Link, Google Scholar
45. : Psychopathy as a disorder of the moral brain: fronto-temporo-limbic grey matter reductions demonstrated by voxel-based morphometry. Neuroimage 2008; 40:1202–1213Crossref, Medline, Google Scholar
46. : Psychopathy and the posterior hippocampus. Behav Brain Res 2001; 118:187–193Crossref, Medline, Google Scholar
47. : Gray matter changes in right superior temporal gyrus in criminal psychopaths: evidence from voxel-based morphometry. Psychiatry Res 2008; 163:213–222Crossref, Medline, Google Scholar
48. : Increased volume of the striatum in psychopathic individuals. Biol Psychiatry 2010; 67:52–58Crossref, Medline, Google Scholar
49. : Mesolimbic dopamine reward system hypersensitivity in individuals with psychopathic traits. Nat Neurosci 2010; 13:419–421Crossref, Medline, Google Scholar
50. : A cognitive developmental approach to morality: investigating the psychopath. Cognition 1995; 57:1–29Crossref, Medline, Google Scholar