Dysregulation of Arousal and Amygdala-Prefrontal Systems in Paranoid Schizophrenia
Abstract
OBJECTIVE: The authors investigated impaired differentiation of limbic-prefrontal systems by autonomic arousal in schizophrenia. It was predicted that paranoid patients would be distinguished by a disjunction of hyperarousal but reduced amygdala and medial prefrontal activity relative to both healthy comparison subjects and patients with nonparanoid schizophrenia. METHOD: Pictures depicting facial expressions of fear were presented to 27 schizophrenia patients (13 paranoid, 14 nonparanoid) and 22 matched healthy comparison subjects in an implicit perception task to evoke limbic activity. Simultaneous functional magnetic resonance imaging and skin conductance arousal recordings were acquired during presentation of faces expressing fear or neutral emotion. Responses to fear stimuli were further examined by contrasting those that were associated with a skin conductance response (“with arousal”) and those that were not (“without arousal”). RESULTS: In the comparison subjects, arousal dissociated amygdala/medial prefrontal (“visceral”) networks and hippocampus/lateral prefrontal (“context”) networks for fear perception. Excessive arousal responses were elicited in the schizophrenia subjects, but there was an associated reduction in amygdala/medial prefrontal activity. This disjunction was pronounced in paranoid patients relative to both healthy subjects and nonparanoid patients. Paranoid patients also showed a relatively greater prefrontal deficit for “without-arousal” responses. CONCLUSIONS: This is the first study to reveal a functional disconnection in autonomic and central systems for processing threat-related signals in patients with paranoid schizophrenia. Paranoid cognition may reflect an internally generated cycle of misattribution regarding incoming fear signals due to a breakdown in the regulation of these systems.
There is a convergence of evidence from neuropsychological, psychophysiological, and neuroimaging studies that breakdowns in temporolimbic-prefrontal circuits are central to the expression of schizophrenia (1–4). Limbic-prefrontal systems show a reciprocal modulatory relationship with autonomic (“body”) arousal via connections with brainstem arousal circuits (5, 6). Abnormalities in both tonic and phasic autonomic arousal have been observed in chronic, first-episode, and high-risk samples (7, 8). We investigated limbic-prefrontal and arousal dysfunction in schizophrenia during perception of facial expressions. A growing number of studies have observed that impairments in facial emotion perception in schizophrenia patients are most pronounced for threat-related expressions such as fear (9, 10). In healthy subjects, fear stimuli typically evoke limbic and prefrontal activity, with preferential engagement of the amygdala (11–14).
In a previous functional magnetic resonance imaging (fMRI) study of healthy subjects, we used simultaneous recording of skin conductance responses to examine the differentiation of limbic-prefrontal systems by autonomic arousal (14). Amygdala and medial prefrontal activity was associated specifically with fear stimuli that evoked a phasic skin conductance arousal response. This pattern of activity may represent a “visceral” system subserving the subjective appraisal of threat (15–19). By contrast, distinct hippocampus lateral prefrontal activity was elicited by stimuli that did not evoke arousal responses and may represent a “context” system for integrating the declarative context of emotionally significant stimuli (16, 19, 20). To date, schizophrenia deficits in the engagement of these limbic-prefrontal circuits have not been examined in relation to autonomic arousal.
In this study, we applied the technique for simultaneous fMRI and skin conductance recordings in a comparison of schizophrenia patients and a larger group of healthy comparison subjects. Schizophrenia was considered in terms of paranoid and nonparanoid subgroups, given our previous observation that neural responses to fear differ across these subtypes (21). Our predictions drew on neurophysiological evidence for a disjunction in autonomic and central responses to emotion in schizophrenia (7). We hypothesized that schizophrenia patients would show dysregulation most apparent in the visceral system: abnormally enhanced arousal (reflecting a heightened autonomic sensitivity to fear) but reduced activity in amygdala-medial prefrontal regions. Given the threat-related emotional content of paranoia, we predicted that this disjunction would distinguish paranoid patients in particular.
Method
Subjects
Twenty-seven schizophrenia outpatients (mean age=27.3 years, SD=9.6) and 22 healthy comparison subjects (mean age=27.2 years, SD=8.1) matched on age and sex distribution (schizophrenia patients: 17 men and 10 women; comparison subjects: 14 men and eight women) took part. Diagnoses of schizophrenia were based on the Composite International Diagnostic Interview (22) and consensus by three psychiatrists (two independent from the study), according to DSM-IV criteria. Exclusion criteria for both groups were left-handedness, neurological disorder or head injury, mental retardation, and meeting DSM-IV criteria for drug dependence as assessed with the Composite International Diagnostic Interview Section M and the Westmead Hospital Clinical Information Base (23). Comparison subjects were also screened for history of a psychiatric illness (in themselves or a first-degree relative) or treatment with psychiatric medication. Schizophrenia and comparison subjects did not differ significantly in terms of mean IQ, indexed by estimated IQ derived from New Adult Reading Test (24) errors (comparison subjects: mean=114.3 [SD=9.4]; schizophrenia patients: mean=110.07 [SD=4.1]).
Mean duration of illness for schizophrenia patients was 4.1 years (SD=2.8). All patients were receiving atypical medication (risperidone [N=14], olanzapine [N=7], clozapine [N=5], or quetiapine [N=1]), and the mean daily dose (in chlorpromazine equivalents [25]) was 356.5 mg (SD=261.1). Schizophrenia symptoms were rated with the Positive and Negative Syndrome Scale (26). Converging assessment served to delineate subgroups. The “paranoid” subgroup (N=13) was defined by a profile of high scores (>3) on four of the positive items of the Positive and Negative Syndrome Scale (delusions, suspiciousness, grandiosity, and excitement), whereas the “nonparanoid” subgroup (N=14) was defined by comparatively low scores (≤3) on these items. Independent-group t tests (corrected alpha=0.003) confirmed that paranoid and nonparanoid groups differed significantly on these four items (Table 1). By contrast, they did not differ significantly on the remaining Positive and Negative Syndrome Scale positive items (hallucinations, conceptual disorganization, hostility) nor on Positive and Negative Syndrome Scale negative items (Table 1). In addition, paranoid and nonparanoid subgroups were comparable in age, sex distribution, chlorpromazine equivalent dosage, New Adult Reading Test errors, and scores on the general psychopathology subscale of the Positive and Negative Syndrome Scale (Table 1). Paranoid patients also met the DSM-IV criteria for the paranoid subtype of schizophrenia.
After complete description of the study, each subject provided written informed consent in accordance with National Health and Medical Research Council ethical guidelines.
Behavioral Task
The experimental paradigm followed that of Williams et al. (14). Subjects viewed standardized gray-scale pictures depicting facial expressions of fear or neutral emotion. Four blocks of fearful expressions alternated with four blocks of neutral expressions, and block order was counterbalanced. Within blocks, eight individual faces were presented randomly for 3 seconds with a 0.75-second interstimulus interval. Subjects carried out a sex classification task for each face, so that the effect of fear was incidental. After scanning, subjects identified the emotion on each face by selecting from seven emotion labels (fear, disgust, anger, sadness, happiness, surprise, neutral expression).
Imaging Protocols
During the behavioral paradigm, 64 T2-weighted images depicting contrasts in blood-oxygen-level-dependent (BOLD) responses were acquired by using a Siemens 1.5-T VISION Plus system at 18 axial 6-mm slices (gap=0.6 mm) parallel to the intercommissural line (TE=40 msec, TR=3 seconds, matrix=128×128).
Data for skin conductance arousal responses were acquired simultaneously by using a customized electrodermal skin conductance system (14), which did not require postacquisition data filtering. Silver-silver chloride electrodes with 0.05 M sodium chloride gel were placed on the distal phalanges of digits II and III of the left hand.
Data Analysis
The methods used for fMRI analysis have been described elsewhere in detail (14, 27). Following motion correction, the experimental design was convolved with two Poisson functions representing hemodynamic delays of 4 and 8 seconds, and the best fit (least-squares) to the weighted sum of these two convolutions was computed at each voxel. The ratio (SSQratio) of the sums of squares attributable to the fitted time series (SSQfit) versus the residuals (SSQresid) was calculated. SSQfit was randomly permuted to provide 10 estimates of SSQratio at each voxel and combined over all voxels to give the null distribution (27). Observed and permuted SSQratio statistic maps were then transformed into standard space. Median SSQratio maps for each group were constructed at the p<0.001 level of significance. Between-group differences in activation were estimated by fitting a one-way analysis of variance (ANOVA) model to generate a map of the main effect of group at each voxel. This map was thresholded to generate a set of spatially contiguous three-dimensional clusters of supratheshold voxel statistics, and the sum of these statistics in each cluster (minimum of 3 voxels) was tested against its permuted null distribution at p<0.01 (two-tailed).
Skin conductance response data were scored by using a sigmoid-exponent model that represents the skin conductance response in mathematical form (28). Skin conductance responses were defined as unambiguous (>0.05 μS) increases in skin conductance with respect to prestimulus baseline, 1–3 seconds poststimulus (29, 30). The equivalent time course of the BOLD and skin conductance responses made it feasible to extract concurrent brain and arousal responses to individual face stimuli. To examine fMRI BOLD responses in relation to skin conductance responses, we first formed two subsets of fear stimuli for each subject, referred to as “with-arousal” and “without-arousal” stimuli. “With-arousal” fear stimuli were those in which the onset of a phasic skin conductance response had occurred within the 3-second stimulus duration. “Without-arousal” stimuli were those that did not elicit a skin conductance response within this period (14).
The schizophrenia and healthy groups were first compared in terms of averaged activity for fearful versus neutral expressions by using the aforementioned fMRI analysis. They were then compared in terms of subaveraged activity contrasts for “with-arousal” versus “without-arousal” responses to facial expressions of fear using the same analytic procedure. Parallel contrasts compared paranoid and nonparanoid schizophrenia subgroups to comparison subjects and to each other. ANOVAs (with expression [fearful versus neutral] as a within-subject factor and diagnosis as a between-group factor) and t tests were used to analyze behavioral and skin conductance response data.
Results
Schizophrenia Versus Comparison Subjects
Recognition accuracy and skin conductance response
Emotion recognition accuracy was generally and significantly impaired in the schizophrenia patients (fear: mean=57% correct [SD=23%]; neutral: mean=65% correct [SD=19%]) relative to the comparison subjects (fear: mean=78% correct [SD=21]; neutral: mean=83% correct [SD=14]) (F=5.2, df=1, 47, p<0.0001). There was no significant group-by-emotion (fear versus neutral) interaction. Differences in recognition also did not covary with either New Adult Reading Test errors or chlorpromazine equivalent measures of medication.
Schizophrenia subjects produced significantly more skin conductance responses than comparison subjects following presentation of facial expressions depicting both fear (t=2.8, df=47, p=0.008) and neutral emotion (t=3.6, df=47, p=0.001) (Figure 1). In within-group analyses, expressions of fear were distinguished from neutral expressions in the comparison subjects by more frequent skin conductance responses (t=3.2, df=21, p=0.005). For schizophrenia subjects, there was a relatively greater amplitude of skin conductance response to expressions of fear, but the difference was nonsignificant (t=1.7, df=26, p=0.09) (Figure 1).
BOLD response
In averaged contrasts for facial expressions of fear relative to neutral emotion, comparison subjects showed significantly increased bilateral BOLD responses in the amygdala and significant increases in the hippocampus, medial prefrontal cortex (extending to the anterior cingulate gyrus and lateral prefrontal cortex), thalamus, and visual cortices (most prominent in bilateral fusiform gyri) (Figure 2 [images A and B], Table 2).
Schizophrenia patients showed significantly reduced activity relative to the comparison subjects in the right amygdala, related central gray region, both medial and lateral prefrontal cortices, and bilateral fusiform gyri (Figure 2 [images C and D], Table 2). Left amygdala activity was also comparatively reduced at a level that approached significance (p=0.06).
In subaveraged contrasts for “with-arousal” expressions of fear (i.e., those eliciting a skin conductance response within the 3-second stimulus duration), significant activity in comparison subjects was localized to the left amygdala and medial prefrontal cortex, extending to the anterior cingulate gyrus (Figure 2 [images E and F], Table 2). Activity was also observed in the central gray and middle temporal regions connected to the amygdala and in the primary motor cortex (Table 2). By contrast, significant activity following “without-arousal” expressions of fear (i.e., those that did not elicit a skin conductance response within the 3-second stimulus duration) was restricted to the right hippocampus and lateral prefrontal cortex, with additional activity in the visual processing stream, including the thalamus and superior temporal gyrus (Figure 2 [images G and H], Table 2).
Schizophrenia patients showed significantly lower activity in the specific “with-arousal” regions observed in the comparison subjects: bilateral amygdala, related central gray and middle temporal areas, and medial prefrontal cortex (Figure 2 [images I and J], Table 2). An additional reduction in the visual (cuneus) region was also observed (Table 2). “Without-arousal” responses showed a less specific pattern of reduced activity in the superior temporal gyrus, thalamus, postcentral gyrus (secondary somatosensory cortex), and visual (inferior parietal/temporal) regions (Figure 2 [images K and L], Table 2).
There were no significant correlations between regions of reduced activity and either New Adult Reading Test errors or chlorpromazine equivalent dosage in schizophrenia subjects for averaged analyses or the subaveraged “with-arousal” and “without-arousal” analyses. In these analyses, there were also no regions in which schizophrenia subjects showed greater activity than comparison subjects.
Paranoid Versus Nonparanoid Subgroups
Recognition accuracy and skin conductance response
There was a significant interaction (F=11.1, df=1, 25, p=0.003) between group (paranoid versus nonparanoid) and emotional expression (fear versus neutral) that was due to the relatively greater impairment for recognition of fear in paranoid patients (Table 1). These differences did not covary with either New Adult Reading Test errors or chlorpromazine equivalent dosage.
Fear stimuli evoked a greater number of skin conductance responses for paranoid than nonparanoid subjects (t=4.0, df=24, p=0.001) (Figure 1). Mean skin conductance response amplitude for fear was also greater for paranoid subjects (t=2.1, df=24, p<0.05) (Figure 1). By contrast, paranoid and nonparanoid patients did not differ in either number of amplitude or skin conductance responses to neutral faces.
BOLD response
In averaged contrasts for facial expressions of fear relative to neutral emotion (Figure 3 [images A–D], Table 3), paranoid subjects showed significantly reduced activity, relative to comparison subjects, in the amygdala and related central gray area, anterior cingulate region of the medial prefrontal cortex, and visual regions (including the fusiform gyrus). The dorsal portion of the medial prefrontal cortex and the visual regions were also areas of significant reduction compared with nonparanoid patients. By contrast, nonparanoid patients showed a significant reduction, relative to comparison subjects, in the hippocampal gyrus and showed no reductions in the amygdala. Nonparanoid subjects were also significantly impaired relative to comparison subjects in the anterior cingulate region of the medial prefrontal cortex but were distinguished from paranoid patients by a greater impairment in the ventral portion of this region.
In subaveraged contrasts for “with-arousal” responses (Figure 3 [images E–H], Table 3), paranoid patients showed reduced activity, relative to comparison subjects, in the amygdala, related central gray area, and medial prefrontal/anterior cingulate cortex. The reduction in amygdala activity was also apparent relative to nonparanoid patients. While nonparanoid patients also showed a “with-arousal” reduction, relative to comparison subjects, in the medial prefrontal cortex, it was only the additional reduction in cerebellar activity that was reduced relative to paranoid patients.
For “without-arousal” responses (Figure 3 [images I–L], Table 3), paranoid patients showed reduced activity relative to comparison subjects in the lateral prefrontal cortex and superior temporal gyrus. The lateral prefrontal reduction was also the region of significant reduction compared with nonparanoid patients. Nonparanoid patients showed a similar reduction, relative to comparison subjects, in the superior temporal gyrus, but they did not differ from paranoid patients in this region. The nonparanoid group was reduced compared with paranoid patients only in the visual (cuneus) region.
Discussion
Consistent with previous findings (11–14), implicit processing of fearful faces in healthy subjects was subserved by activity in limbic, prefrontal, and visual brain regions. Skin conductance arousal responses differentiated distinct “with-arousal” amygdala/medial prefrontal activity from “without-arousal” hippocampus/lateral prefrontal activity. Amygdala activity was also associated with responses in the anatomically connected central gray region, which may reflect functional projections to the autonomic networks via the brainstem (6, 15). These networks accord with our earlier finding, in a smaller sample, that arousal dissociates a “visceral” from “context” system (14). Medial prefrontal involvement may allow for the cognitive appraisal of visceral input and subsequent decision making (18, 19). By contrast, the lateral prefrontal cortex receives considerable innervation from the hippocampus (with only meager amygdala connections) and may subserve processing of stimulus context in working memory (16, 20).
Schizophrenia impairments were due primarily to a dysfunction of the visceral network, which was most pronounced in paranoid patients. Notably, paranoid patients produced excessive arousal responses relative to both comparison subjects and to nonparanoid patients, suggesting a heightened autonomic responsivity to threat-related signals in this group. By contrast, arousal responses for nonparanoid patients were similar to those for healthy comparison subjects. Despite enhanced arousal, paranoid patients showed a reduction in “with-arousal” amygdala, central gray area, and dorsomedial prefrontal activity. In these patients, there was a particularly marked reduction in “with-arousal” amygdala activity relative to both healthy subjects and nonparanoid patients.
Nonparanoid schizophrenia patients also showed a reduction in “with-arousal” medial prefrontal activity (in this case, the ventral portion) but were distinguished by a “with-arousal” reduction in cerebellar, rather than amygdala, activity. There was a further distinctive reduction in hippocampal gyrus activity for nonparanoid patients in the general averaged response to fear. This pattern suggests that nonparanoid schizophrenia impairments in emotion perception do not reflect a specific dysfunction of the visceral network. Rather, they may be due to a general inability to coordinate and contextualize salient stimuli via cerebellar-hippocampal-prefrontal circuits, which is independent of any abnormalities in feedback from autonomic arousal. This proposal accords with neurophysiological evidence that the failure to contextualize task-relevant signals is apparent in frontal brain regions in negative symptom schizophrenia during periods of phasic arousal (23).
For “without-arousal” stimuli, schizophrenia patients as a group did not show specific reductions in the context (hippocampus/lateral prefrontal) network. However, paranoid patients were distinguished by a reduction in the lateral prefrontal cortex (extending medially) relative to both healthy subjects and to nonparanoid patients. This reduction suggests that paranoid patients may have an additional deficit in the integration of threat-related signals in working memory. This deficit may reflect a consequence of the ineffective processing of these incoming signals via the visceral network. Both paranoid and nonparanoid patients showed an impairment in “without-arousal” superior temporal gyrus function, consistent with the impairment in general face and emotion processing observed in these patients (13).
Taken together, the findings suggest that paranoid schizophrenia is characterized by a specific disjunction of arousal and amygdala-prefrontal circuits that leads to impaired processing of significant, particularly threat-related, signals. The pattern of excessive arousal but reduced amygdala activity in paranoid patients points to a dysregulation in the normal cycle of mutual feedback between amygdala function and somatic state (autonomic activity). The concomitant lack of “with-arousal” medial prefrontal engagement suggests that this region cannot undertake its usual role in regulating amygdala-autonomic function, leading to a perseveration and exacerbation of arousal responses (18, 19). A functional breakdown of autonomic-amygdala-prefrontal systems could readily lead to an internally generated cycle of hypervigilance and misattribution that feeds into paranoid cognition (31).
Given evidence for habituation of amygdala responses (32, 33), group differences in habituation might be considered as an alternative account of the present findings. However, the abnormally high number and magnitude of skin conductance responses in schizophrenia subjects, and their spread across all trials and face stimuli, indicated that we might discount a simple hypothesis of greater amygdala habituation in these individuals. Previous skin conductance response studies have also reported a comparative failure of habituation in schizophrenia and in “at-risk” samples (34, 35).
Combined functional and structural imaging studies are warranted to explore the possible role of structural deficits (1) in these limbic-prefrontal disturbances. Consideration of more specifically defined syndromes and gender differences with a larger sample is also warranted, given evidence from neuroimaging, neuropsychology, and neurophysiology studies that brain function and structure differ across both sex and symptom profile (1, 23, 36). In addition, this study demonstrates the value of the concurrent fMRI-skin conductance response technique for future investigations of treatment in schizophrenia. The arousal-inhibiting effect of atypical antipsychotic treatments was a key factor in initiating their development, and these agents are designed to more specifically target dopamine receptors in the limbic system (37). The approach employed in this study provides a means to study both the arousal and limbic-prefrontal effects of atypical medications within a single paradigm.
![]() |
![]() |
![]() |
Received Feb. 11, 2003; revision received July 3, 2003; accepted July 10, 2003. From The Brain Dynamics Centre, Westmead Hospital and University of Sydney. Address reprint requests to Dr. Williams, The Brain Dynamics Centre, Acacia House, Westmead Hospital, Westmead, NSW 2145, Australia; [email protected] (e-mail). This study was supported by research grant funding from Janssen-Cilag, the Neuroscience Institute of Schizophrenia & Allied Disorders, Rebecca Cooper Medical Research Foundation, the University of Sydney (School of Psychology and a Sesqui award), and the Wellcome Trust Collaborative Biomedical (Ref: 058031/Z/99/Z).
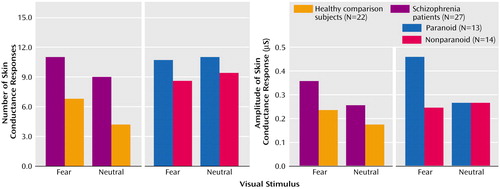
Figure 1. Skin Conductance Responses to Pictures Depicting Facial Expressions of Fear or Neutral Emotion in Patients With Paranoid or Nonparanoid Schizophrenia and Healthy Comparison Subjects
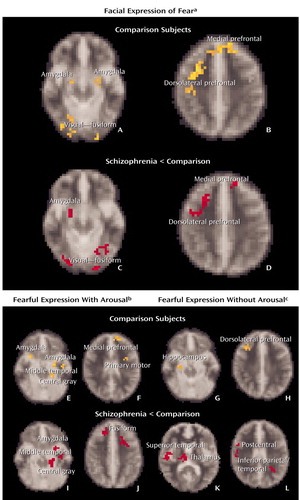
Figure 2. Regional Activation Maps of BOLD Response to Pictures Depicting Facial Expressions of Fear Versus Neutral Emotion in Schizophrenia Patients (N=27) and Healthy Comparison Subjects (N=22)
aResponse relative to that seen following presentation of neutral facial expression.
bPicture elicited a skin conductance response within the 3-second stimulus duration.
cPicture did not elicit a skin conductance response within the 3-second stimulus duration.
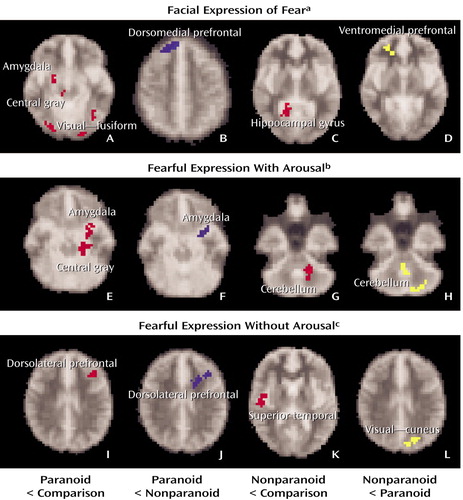
Figure 3. Regional Activation Maps of Significant Differences in BOLD Response to Pictures Depicting Facial Expressions of Fear Versus Neutral Emotion in Patients With Paranoid (N=13) or Nonparanoid (N=14) Schizophrenia and Healthy Comparison Subjects (N=22)
aResponse relative to that seen following presentation of neutral facial expression.
bPicture elicited a skin conductance response within the 3-second stimulus duration.
cPicture did not elicit a skin conductance response within the 3-second stimulus duration.
1. Gur RE, Turetsky BI, Cowell PE, Finkelman C, Maany R, Grossman R, Arnold S, Bilker W, Gur RC: Temporolimbic volume reductions in schizophrenia. Arch Gen Psychiatry 2000; 57:769–775Crossref, Medline, Google Scholar
2. Hill SK, Ragland JD, Gur RC, Gur RE: Neuropsychological differences among empirically derived clinical subtypes of schizophrenia. Neuropsychology 2001; 15:492–501Crossref, Medline, Google Scholar
3. Bogerts B, Lieberman JA, Ashtari M, Bilder RM, Degreef G, Lerner G, Johns C, Masiar S: Hippocampus-amygdala volumes and psychopathology in chronic schizophrenia. Biol Psychiatry 1993; 33:236–246Crossref, Medline, Google Scholar
4. Flor-Henry P: Lateralized temporal-limbic dysfunction and psychopathology. Ann NY Acad Sci 1976; 180:777–797Crossref, Google Scholar
5. LeDoux E: The Emotional Brain. London, Weidenfeld Nicolson, 1998Google Scholar
6. Gray JA: The Psychology of Fear and Stress, vol 2. New York, Cambridge University Press, 1987Google Scholar
7. Kring AM, Neale JM: Do schizophrenic patients show a disjunctive relationship among expressive, experiential, and psychophysiological components of emotion? J Abnorm Psychol 1996; 105:249–257Crossref, Medline, Google Scholar
8. Lindstrom EM, Ohlund LS, Lindstrom LH, Ohman A: Symptomatology and electrodermal activity as predictors of neuroleptic response in young male schizophrenic patients. Psychiatry Res 1992; 42:145–158Crossref, Medline, Google Scholar
9. Mandal MK, Pandey R, Prasad AB: Facial expressions of emotion and schizophrenia: a review. Schizophr Bull 1998; 24:399–412Crossref, Medline, Google Scholar
10. Edwards J, Jackson JH, Pattison PE: Emotion recognition via facial expression and affective prosody in schizophrenia: a methodological review. Clin Psychol Rev 2002; 22:789–832Crossref, Medline, Google Scholar
11. Phillips ML, Young AW, Senior C, Calder AJ, Rowland D, Brammer M, Bullmore ET, Andrew C, Williams SCR, Gray J, David A: A specific neural substrate for perceiving facial expressions of disgust. Nature 1997; 389:495–498Crossref, Medline, Google Scholar
12. Morris JS, Frith CD, Perrett DI, Rowland D, Young AW, Calder AJ, Dolan RJ: A differential neural response in the human amygdala to fearful and happy facial expressions. Nature 1986; 383:812–815Crossref, Google Scholar
13. Phan KL, Wager T, Taylor SF, Liberzon I: Functional neuroanatomy of emotion: a meta-analysis of emotion activation studies in PET and fMRI. Neuroimage 2001; 16:331–348Crossref, Google Scholar
14. Williams LM, Phillips ML, Brammer MJ, Skerrett D, Lagopoulos J, Oliveri G, Bahramali H, David AS, Peduto A, Gordon E: Arousal dissociates amygdala and hippocampal fear responses: evidence from simultaneous fMRI and skin conductance responses. Neuroimage 2001; 14:1070–1079Crossref, Medline, Google Scholar
15. An X, Bandler R, Öngür D, Price JL: Prefrontal cortical projections to longitudinal columns in the midbrain periaqueductal gray in macaque monkeys. J Comp Neurol 1998; 401:455–479Crossref, Medline, Google Scholar
16. Bechara A, Tranel D, Damasio H, Adolphs R, Rockland C, Damasio AR: Double dissociation of conditioning and declarative knowledge relative to the amygdala and hippocampus in humans. Science 1995; 269:1115–1118Crossref, Medline, Google Scholar
17. Wehner JM, Radcliffe RA, Rosmann ST, Christensen SC, Rasmussen DL, Fulker DW, Wiles M: Quantitative trait locus analysis of contextual fear conditioning in mice. Nat Genet 1997; 17:331–334Crossref, Medline, Google Scholar
18. Damasio AR: The somatic marker hypothesis and the possible functions of the prefrontal cortex. Phil Trans R Soc Lond B 1996; 351:1413–1420Crossref, Medline, Google Scholar
19. Drevets WC, Raichle ME: Reciprocal suppression of regional cerebral blood flow during emotional versus higher cognitive processes: implications for interactions between emotion and cognition. Cognition and Emotion 1998; 12:353–385Crossref, Google Scholar
20. Goldman-Rakic PS: Topography of cognition: parallel distributed networks in primate association cortex. Ann Rev Neurosci 1998; 11:137–156Crossref, Google Scholar
21. Phillips ML, Williams L, Senior C, Bullmore ET, Brammer MJ, Andrew C, Williams SCR, David AS: A differential neural response to threatening and non-threatening negative facial expressions in paranoid and nonparanoid schizophrenics. Psychiatr Res Neuroimaging 1999; 92:11–31Crossref, Medline, Google Scholar
22. Robins LN, Wing J, Wittchen HU, Helzer JE, Babor TF, Burke J, Farmer A, Jablenski A, Pickens R, Regier DA, Sartorius N, Towle LH: The Composite International Diagnostic Interview: an epidemiologic instrument suitable for use in conjunction with different diagnostic systems and in different cultures. Arch Gen Psychiatry 1988; 45:1069–1077Crossref, Medline, Google Scholar
23. Williams LL, Bahramali H, Hemsley DR, Harris AWF, Brown K, Gordon E: Electrodermal responsivity distinguishes ERP activity and symptom profile in schizophrenia. Schizophr Res 2003; 59:115–125Crossref, Medline, Google Scholar
24. Nelson HE, O’Connell A: Dementia: the estimation of premorbid intelligence levels using the New Adult Reading Test. Cortex 1978; 14:234–244Crossref, Medline, Google Scholar
25. van Kammen DP, Marder SR: Dopamine receptor antagonists, in Comprehensive Textbook of Psychiatry, 6th ed, vol 2. Edited by Kaplan HI, Sadock BJ. Baltimore, Williams & Wilkins, 1995, pp 1987–2022Google Scholar
26. Kay SR, Opler LA, Fiszbein A: Positive and Negative Syndrome Scale (PANSS). North Tonawanda, NY, Multi-Health Systems, 1986Google Scholar
27. Brammer MJ, Bullmore ET, Simmons A, Williams SCR, Grasby PM, Howard RJ, Woodruff PWR, Rabe-Hesketh S: Generic brain activation mapping in functional magnetic resonance imaging: a nonparametric approach. Magn Res Imag 1997; 15:763–770Crossref, Medline, Google Scholar
28. Lim CL, Rennie C, Barry JR, Bahramali H, Lazzaro I, Manor B, Gordon E: Decomposing skin conductance into tonic and phasic components. Int J Psychophysiol 1997; 25:97–109Crossref, Medline, Google Scholar
29. Boucsein W: Electrodermal Activity. New York, Plenum, 1992Google Scholar
30. Barry RJ, Sokolov EN: Habituation of phasic and tonic components of the orienting reflex. Int J Psychophysiol 1993; 15:30–42Google Scholar
31. Phillips ML, Senior C, David AS: Perception of threat in schizophrenics with persecutory delusions: an investigation using visual scan paths. Psychol Med 2000; 30:157–167Crossref, Medline, Google Scholar
32. Phillips ML, Medford N, Young AW, Williams L, Williams SC, Bullmore ET, Gray JA, Brammer MJ: Time courses of the left and right amygdalar responses to fearful facial expressions. Hum Brain Mapp 2001; 12:193–202Crossref, Medline, Google Scholar
33. Brieter HC, Ectoff NL, Whalen PJ, Kennedy WA, Rauch SL, Petersen SE, Raichle ME, Rosen BR: Response and habituation of the human amygdala during visual processing of facial expression. Neuron 1996; 17:875–887Crossref, Medline, Google Scholar
34. Schiffer RA, Sigal M, Mintz M: Delayed habituation of the skin-conductance orienting response correlates with impaired performance on the Wisconsin Card Sorting Task in schizophrenia. Psychiatry Res 1996; 65:107–112Crossref, Medline, Google Scholar
35. Hollister JM, Mednick SA, Brennan P, Cannon TD: Impaired autonomic nervous system-habituation in those at genetic risk for schizophrenia. Arch Gen Psychiatry 1994; 51:552–558Crossref, Medline, Google Scholar
36. Liddle PF: Syndromes in schizophrenia and their neuropsychological and neuroanatomical correlates, in Schizophrenia: A Neuropsychological Perspective. Edited by Pantelis C, Nelson HE, Barnes TRE. Chichester, UK, John Wiley & Sons, 1996, pp 299–316Google Scholar
37. Worrel JA, Marken PA, Beckman SE, Ruehter VL: Atypical antipsychotic agents: a critical review. Am J Health Syst Pharm 2000; 57:238–258Crossref, Medline, Google Scholar