Catechol O-Methyltransferase Val158Met Polymorphism in Schizophrenia: Differential Effects of Val and Met Alleles on Cognitive Stability and Flexibility
Abstract
OBJECTIVE: The catechol O-methyltransferase (COMT) Val158Met polymorphism has been associated with cognitive and behavioral phenotypes in schizophrenia. Whether COMT genotype is beneficial may depend on phenotype definition. The authors examined the effects of COMT genotype on a task that distinguishes imitation from reversal learning. They hypothesized that the Val and Met alleles would be associated with deficits in imitation learning and reversal learning, respectively. METHOD: Twenty-six patients with schizophrenia and schizoaffective disorder completed a task requiring alternation between imitation and reversal rules. RESULTS: Met homozygotes showed better acquisition of the imitation rule but greater deficit shifting from imitation to reversal. Val homozygotes had poorer imitation performance and slower reaction times. CONCLUSIONS: The Met allele, by increasing tonic dopamine, may promote cognitive stability but limit cognitive flexibility.
Catechol O-methyltransferase (COMT) is an important enzyme in dopamine metabolism. A common functional polymorphism at codon 158 in the gene coding for COMT (COMT Val158Met) results in substantial effects, with homozygosity for the low-activity (Met) allele leading to a three- to fourfold reduction in enzymatic activity compared with the high-activity (Val) allele. This polymorphism has been associated with diverse phenotypes in patients with schizophrenia. The Met allele has been associated with aggressive/dangerous behavior (e.g., references 1, 2) but also with better performance on cognitive tasks (3) and increased prefrontal efficiency (4). Bilder et al. (3) further report that symptom ratings were higher in Met homozygotes despite better neurocognitive scores.
These complex associations are difficult to explain by a unitary hypothesis of increased prefrontal dopamine. Manipulations of dopamine can be beneficial or detrimental (5, 6). The tonic/phasic dopamine theory (7–9) may help resolve these apparent discrepancies. Phasic dopamine may be important for updating or resetting working memory traces, principally via D2 receptors, while tonic dopamine may enhance stability of traces, principally via D1 receptors (10). Detailed biophysical models suggest that sustained D1 activation (tonic dopamine) helps prevent “uncontrolled, spontaneous switches into high-activity states (i.e., spontaneous activation of task-irrelevant representations)” (11, 12).
Since COMT catabolizes extracellular dopamine, while rapid intrasynaptic degradation relies on the dopamine transporter (phasic dopamine), the Met allele may enhance tonic dopamine but have reciprocal effects on phasic dopamine. We hypothesized that the Met allele would be of benefit for tasks requiring tonic activation (cognitive stability) but have adverse effects on tasks requiring phasic activation (cognitive flexibility).
Most tasks used in studies of COMT genotype have demands for both cognitive stability and flexibility. The Wisconsin Card Sorting Test requires cognitive stability to establish and maintain an appropriate response set and flexibility for the set-shifting aspect of the task. N-back working memory tasks require both maintenance (stability) and updating (flexibility) of memory representations.
In this study we used a computerized Competing Programs Task (13) that requires alternation between two rules of responding—imitation and reversal. Acquisition and maintenance of the imitation rule requires cognitive stability, whereas cognitive flexibility is needed to switch rules in the reversal condition.
Method
Twenty-six subjects (18 men and eight women; 25 white, one Hispanic; mean age=41.4 years [range=30–52]) with schizophrenia (N=21) or schizoaffective disorder (N=5) completed the Competing Programs Task (13). Each subject provided written informed consent after receiving a complete description of the study, according to the guidelines of the Institutional Review Board. At the time of testing, subjects were clinically diagnosed, stable inpatients being treated with a variety of antipsychotic medications sometimes in combination with adjunctive mood stabilizers or antidepressants. Severity of symptoms was not formally assessed at the time of testing.
The Competing Programs Task requires subjects to respond with one or two key presses after seeing one or two visual/auditory cues (one or two squares presented simultaneously with the same number of tones). During blocks 1 and 3 (imitation), the subject must produce the same number of key presses as stimuli; during blocks 2 and 4 (reversal) the subject must press once for two stimuli and twice for a single stimulus. Each block ends (and the rule changes) after either a maximum of 20 trials or a criterion (eight consecutive correct responses) is reached. The subject must deduce the response rules from trial-by-trial feedback. COMT genotyping was performed as described previously (14).
Accuracy measures included trials to criterion and proportion correct. We also calculated a proportional cost index (15) to measure performance decrement related to shifting in the response rules from imitation to reversal: (proportion correct [imitation] – proportion correct [reversal]) / proportion correct [reversal].
Results
There were eight COMT Met homozygotes, six Val homozygotes, and 12 heterozygotes. The effect of genotype on reaction time was significant (F=4.8, df=2, 23, p<0.02; partial η2=0.29); reaction time was slower in Val than Met homozygotes (p<0.05, least significant difference test).
The effect of response rule (imitation/reversal) on proportion correct was significant (F=10.2, df=1, 23, p=0.004; η2=0.31; imitation better than reversal), as was the response rule-by-genotype interaction (F=7.2, df=2, 23, p=0.004; η2=0.39). Met homozygotes had greater accuracy than Val homozygotes for imitation responses but not reversal responses (Figure 1). The response rule-by-genotype interaction was also significant for trials to criterion (F=4.4, df=2, 23, p<0.03; η2=0.28). Trials to criterion was lower for Met homozygotes than Val homozygotes for imitation blocks only (t=–2.2, df=23, p<0.05). There were significant differences between genotypes in proportional cost index (F=7.92, df=2, 23, p=0.002; η2=0.41). Met homozygotes showed the greatest sensitivity to conflict (Figure 1).
Discussion
Consistent with the hypothesis, the Met allele of the COMT Val158Met polymorphism was associated with better cognitive stability but poorer cognitive flexibility. Met homozygotes showed better acquisition of the imitation rule but greater sensitivity to conflict when the response rules shifted from imitation to reversal. Since Met homozygotes had poorer absolute (not just relative) scores in the reversal condition, the increased “cost” of shifting does not appear to be an artifact of better performance in the imitation condition.
These findings suggest it may be plausible to define more precisely the phenotype most relevant to COMT genotype. In previous studies, COMT genotype accounted for 4% (4, 16) to 11% (3) of the variance in performance. In this study, COMT genotype accounted for 28%–41% of the variance in performance on the Competing Programs Task.
Our results are consistent with the hypothesis that the Met allele leads to increased tonic dopamine activity, with general benefits for cognitive stability, but costs in the capacity to flexibly alter behavioral programs. This may help explain how the Met allele can be associated both with better cognitive performance and increased aggression. Further investigation of COMT effects may benefit from incorporating methods distinguishing cognitive stability from plasticity.
While these preliminary results may offer proof of concept, replication is clearly needed. Future research particularly needs to examine larger and more fully characterized samples and to look for possible genotype-by-treatment interactions. Given these caveats, we hope the findings may be useful in future attempts to refine the phenotypes associated with the COMT Val158Met polymorphism.
Received Dec. 4, 2002; revisions received June 3 and July 15, 2003; accepted July 21, 2003. From the Nathan S. Kline Institute for Psychiatric Research. Address reprint requests to Dr. Nolan, Nathan S. Kline Institute for Psychiatric Research, 140 Old Orangeburg Rd., Building 35, Orangeburg, NY 10962.
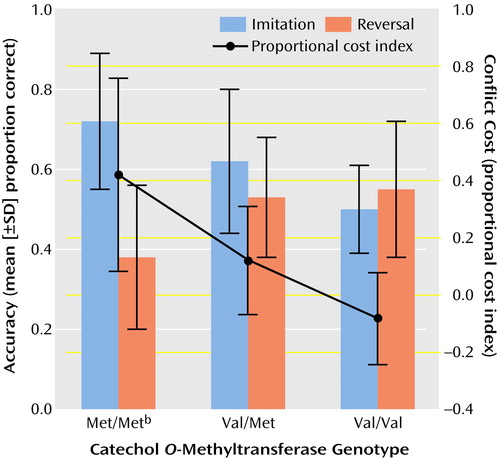
Figure 1. Accuracy and “Conflict Cost” as a Function of COMT Genotype in 26 Patients With Schizophrenia or Schizoaffective Disorder Given the Competing Programs Taska
aSubjects were presented with one or two cues and were asked to follow one of two alternating rules: pressing a key the same number of times as there were cues (imitation) or pressing once for two cues and twice for one cue (reversal). “Conflict cost” refers to the performance decrement related to shifting in the response rules from imitation to reversal; the proportional cost index is calculated as follows: (proportion correct [imitation] – proportion correct [reversal]) / proportion correct (reversal).
bSignificantly greater accuracy than the Val/Val genotype (t=2.5, df=23, p<0.03) but significantly greater sensitivity to conflict (i.e, less cognitive flexibility) than seen with the other two genotypes (F=7.92, df=2, 23, p=0.002).
1. Lachman HM, Nolan KA, Mohr P, Saito T, Volavka J: Association between catechol O–methyltransferase genotype and violence in schizophrenia and schizoaffective disorder. Am J Psychiatry 1998; 155:835–837Abstract, Google Scholar
2. Strous RD, Bark N, Parsia SS, Volavka J, Lachman HM: Analysis of a functional catechol-O–methyltransferase gene polymorphism in schizophrenia: evidence for association with aggressive and antisocial behavior. Psychiatry Res 1997; 69:71–77Crossref, Medline, Google Scholar
3. Bilder RM, Volavka J, Malhotra AK, Kennedy JL, Ni X, Goldman RS, Hoptman MJ, Sheitman B, Lindenmayer JP, Citrome L, McEvoy J, Kunz M, Chakos M, Cooper TB, Lieberman JA: Neurocognitive correlates of the COMT Val158Met polymorphism in chronic schizophrenia. Biol Psychiatry 2002; 52:701–707Crossref, Medline, Google Scholar
4. Egan MF, Goldberg TE, Kolachana BS, Callicott JH, Mazzanti CM, Straub RE, Goldman D, Weinberger DR: Effect of COMT Val108/158 Met genotype on frontal lobe function and risk for schizophrenia. Proc Natl Acad Sci USA 2001; 98:6917–6922Crossref, Medline, Google Scholar
5. Mattay VS, Goldberg TE, Fera F, Hariri AR, Tessitore A, Egan MF, Kolachana B, Callicott JH, Weinberger DR: Catechol O–methyltransferase val158-met genotype and individual variation in the brain response to amphetamine. Proc Natl Acad Sci USA 2003; 100:6186–6191Crossref, Medline, Google Scholar
6. Mehta MA, Swainson R, Ogilvie AD, Sahakian J, Robbins TW: Improved short-term spatial memory but impaired reversal learning following the dopamine D(2) agonist bromocriptine in human volunteers. Psychopharmacology (Berl) 2001; 159:10–20Crossref, Medline, Google Scholar
7. Grace AA: Phasic versus tonic dopamine release and the modulation of dopamine system responsivity: a hypothesis for the etiology of schizophrenia. Neuroscience 1991; 41:1–24Crossref, Medline, Google Scholar
8. Grace AA: Cortical regulation of subcortical dopamine systems and its possible relevance to schizophrenia. J Neural Transm Gen Sect 1993; 91:111–134Crossref, Medline, Google Scholar
9. Moore H, West AR, Grace AA: The regulation of forebrain dopamine transmission: relevance to the pathophysiology and psychopathology of schizophrenia. Biol Psychiatry 1999; 46:40–55Crossref, Medline, Google Scholar
10. Cohen J, Braver T, Brown J: Computational perspectives on dopamine function in prefrontal cortex. Curr Opin Neurobiol 2002; 12:223–229Crossref, Medline, Google Scholar
11. Durstewitz D, Seamans JK: The computational role of dopamine D1 receptors in working memory. Neural Netw 2002; 15:561–572Crossref, Medline, Google Scholar
12. Durstewitz D, Seamans JK, Sejnowski TJ: Dopamine-mediated stabilization of delay-period activity in a network model of prefrontal cortex. J Neurophysiol 2000; 83:1733–1750Crossref, Medline, Google Scholar
13. Bilder RM, Turkel E, Lipschutz-Broch L, Lieberman JA: Antipsychotic medication effects on neuropsychological functions. Psychopharmacol Bull 1992; 28:353–366Medline, Google Scholar
14. Nolan KA, Volavka J, Czobor P, Cseh A, Lachman H, Saito T, Tiihonen J, Putkonen A, Hallikainen T, Kotilainen I, Rasanen P, Isohanni M, Jarvelin MR, Karvonen MK: Suicidal behavior in patients with schizophrenia is related to COMT polymorphism. Psychiatr Genet 2000; 10:117–124Crossref, Medline, Google Scholar
15. Meiran N, Levine J, Meiran N, Henik A: Task set switching in schizophrenia. Neuropsychology 2000; 14:471–482Crossref, Medline, Google Scholar
16. Malhotra AK, Kestler LJ, Mazzanti C, Bates JA, Goldberg T, Goldman D: A functional polymorphism in the COMT gene and performance on a test of prefrontal cognition. Am J Psychiatry 2002; 159:652–654Link, Google Scholar