The Nature of Traumatic Memories: A 4-T fMRI Functional Connectivity Analysis
Abstract
OBJECTIVE: This study used functional connectivity analyses to assess interregional brain activity correlations during the recall of traumatic memories in traumatized subjects with and without posttraumatic stress disorder (PTSD). METHOD: Both 4-T functional magnetic resonance imaging (fMRI) and functional connectivity analyses were used to assess interregional brain activity correlations during script-driven symptom provocation in traumatized subjects with (N=11) and without (N=13) PTSD. Functional connectivity analyses were carried out by using data for brain regions activated in both the PTSD group and the comparison group. The use of functional connectivity analyses in addition to subtraction analyses allowed assessment of specific brain regions involved in the recall of traumatic events and of the neuronal networks underlying the recall of such events. RESULTS: Significant between-group differences in functional connectivity were found. Comparison of connectivity maps at coordinates x=2, y=20, z=36 (right anterior cingulate gyrus) for the two groups showed that the subjects without PTSD had greater correlation than the PTSD subjects in the left superior frontal gyrus (Brodmann’s area 9), left anterior cingulate gyrus (Brodmann’s area 32), left striatum (caudate), left parietal lobe (Brodmann’s areas 40 and 43), and left insula (Brodmann’s area 13). In contrast, the PTSD subjects showed greater correlation than the subjects without PTSD in the right posterior cingulate gyrus (Brodmann’s area 29), right caudate, right parietal lobe (Brodmann’s areas 7 and 40), and right occipital lobe (Brodmann’s area 19). CONCLUSIONS: The differences in brain connectivity between PTSD and comparison subjects may account for the nonverbal nature of traumatic memory recall in PTSD subjects, compared to a more verbal pattern of traumatic memory recall in comparison subjects.
Previous neuroimaging studies of patients with posttraumatic stress disorder (PTSD) have examined specific brain regions involved in the recall of traumatic events and have implicated limbic, paralimbic, and prefrontal structures in the pathophysiology of PTSD (1–10). Paralimbic structures that are thought to underlie PTSD include the anterior cingulate gyrus (Brodmann’s areas 24 and 32) and the orbitofrontal cortex. Prefrontal structures implicated in PTSD include the left inferior prefrontal cortex and Broca’s area (2–6, 11). In support of these findings, our group, by using functional magnetic resonance imaging (fMRI), showed that PTSD patients who had a hyperarousal response to traumatic transcript-driven imagery had significantly less activation in the anterior cingulate gyrus (Brodmann’s area 32), medial prefrontal cortex (Brodmann’s area 11), and thalamus, as compared to traumatized subjects who never met the criteria for PTSD (7).
Abnormal connectivity among regions involved in the recall of traumatic material may also be an important mechanism underlying the particular way in which traumatic memories are experienced by PTSD patients. To our knowledge, PTSD neuroimaging studies have not addressed the functional connectivity underlying the recall of traumatic memories. The type of “subtraction analyses” usually used in PTSD neuroimaging studies are able to delineate specific brain regions involved in the recall of traumatic events; functional connectivity analyses, however, enable the assessment of interregional correlations in brain activity. Given the overlapping activation of paralimbic and subcortical structures across different anxiety disorders (8), such analyses may prove useful in distinguishing the neural networks underlying different anxiety disorders by examining functional connectivity among regions of activation. The purpose of this study was therefore to use functional connectivity analyses to assess interregional brain activity correlations during the recall of traumatic memories in traumatized subjects with and without PTSD. We hypothesized that functional connectivity analyses for the PTSD patients would reveal neuronal networks more consistent with a nonverbal pattern of memory recall and that functional connectivity analyses for the comparison subjects would show neuronal networks more consistent with a verbal pattern of memory recall.
Method
Subjects
Eleven subjects who had developed PTSD as a result of sexual abuse/assault (N=6) or a motor vehicle accident (N=5) were included in the study. Comparison subjects were 13 subjects who met criterion A for PTSD (as a result of sexual abuse/assault [N=5] or motor vehicle accident [N=8]) but who never developed PTSD. Informed consent was obtained from all subjects. PTSD and comparison subjects were assessed by using the Structured Clinical Interview for DSM-IV and the Clinician-Administered PTSD Scale (PTSD subjects: mean=72, SD=14; comparison subjects: mean=4, SD=3) (12). Comorbidity in the PTSD group included current major depression (N=4), current dysthymic disorder (N=3), current panic disorder (N=2), lifetime (past) history of drug abuse/dependence (N=2), lifetime (past) history of alcohol abuse/dependence (N=4), and current nicotine abuse (N=5). The comparison subjects were of similar age (PTSD subjects: mean=36 years, SD=12; comparison subjects: mean=34 years, SD=13), sex, and race and were free of any psychiatric illness. All subjects were right-handed. The patients had undergone a supervised drug washout for at least 2 weeks before scanning. Patients with a history of psychosis, bipolar disorder, and substance use disorder in remission for less than 6 months were excluded from the study, as were patients with any significant medical conditions, neurological illness, or a history of head injury. Nine PTSD subjects and nine comparison subjects included in the analyses for the present paper were also included in previously reported analyses (7).
fMRI Procedures
MR imaging studies were performed on a 4-T whole-body Varian/Siemens imaging system (Palo Alto, Calif./Erlangen, Germany) with a 90-cm diameter horizontal bore and a whole-body 68-cm diameter gradient set with a maximum strength of 40 mT/minute and a slew rate of 120 mT/minute. A whole-head hybrid birdcage radio frequency coil was used for transmission and detection of signals. Before imaging, a global shimming procedure, using first- and second-order shims, was performed to optimize the magnetic field over the imaging volume of interest. The subject’s heart rate was monitored by using a fiber-optic pulse oximeter.
The radio frequency coil was placed around the subject’s head. Each functional brain volume was acquired by using a navigator-echo-corrected, interleaved multishot (four shots) echo-planar imaging pulse sequence with a 64×64 matrix size and a total volume acquisition time of 5 seconds (TE=15 msec, flip angle=45°, field of view=24.0 cm). The volume acquired consisted of 12 transverse slices, each of which was 6 mm thick (voxel size=1.87×1.87×6 mm). Extreme dorsal and ventral territories of the brain were excluded.
Script-Driven Imagery
The script-driven imagery procedure was adapted to fMRI according to previously published methods (7, 13, 14).
Scanning of traumatic and neutral imagery conditions was repeated three times. Each scan proceeded as follows: Each subject was instructed to lie still and allow himself/herself to begin focusing on the script of a traumatic or neutral event that he/she had experienced as soon as the script was read. Reading of the script lasted 30 seconds. As soon as the subject heard the script, he/she was encouraged to remember olfactory, auditory, somatosensory, and visual sensations that were associated with the traumatic event for 60 seconds. Measurements of heart rate occurred during this time. A total of 120 seconds were allowed to pass until the script was repeated. During this time, the subject was asked to lie still, breathe through his/her nose, and let go of the traumatic event. Baseline brain activation was calculated on the basis of the average activation patterns 60 seconds before each recollection of the traumatic event. Brain activation during recall of the traumatic event was calculated on the basis of the average activation patterns during the final 30 seconds of recall of the traumatic event.
Subjects’ responses to the traumatic script-driven imagery were assessed by using a scale developed by Hopper (unpublished 1998 manuscript of J.W. Hopper). This scale assesses PTSD symptom responses and dissociative and emotional responses during the recall of traumatic and neutral scripts.
Autonomic responsivity to script-driven memories was assessed by averaging the change in heart rate from baseline across the three provocations, and t tests were used to compare the responsivity of PTSD patients with that of the comparison subjects.
SPM Analysis
Subtraction analyses (fixed-effects model)
These statistical analyses employed voxel-wise general linear models (15) with design matrices comprising epoch-related regressors. Regional activations attributable to traumatic memory recall for each subject group (PTSD patients and comparison subjects) and between groups were ascertained by using basic subtraction analyses. Linear contrasts were utilized to test within- and between-group hypotheses about significant differences in location and intensity of blood-oxygen-level-dependent (BOLD) response during the script-driven imagery task, relative to BOLD response measured during baseline. These linear contrasts yield statistical parametric maps of the t statistic, SPM(t). The within-group SPM(t) maps were thresholded at p<0.001, corrected for multiple comparisons at the voxel-cluster level of analysis (cluster size=approximately ≥10 voxels), and the between-group SPM(t) maps were thresholded at p<0.05, corrected for multiple comparisons, cluster-wise (voxel number=approximately ≥10). Since, to our knowledge, this study is the first to use psychophysiological interaction analyses in PTSD, we wanted to balance our conservative type I error protection against false negative results. Therefore, the SPM(t) maps were corrected for multiple comparisons and thresholded at p<0.05 in the psychophysiological interaction analyses; the subtraction analyses were thresholded at p<0.001. In doing so, we invoked considerable type I error protection in the (preliminary) subtraction analysis and more than the usual protection against false positive results in the psychophysiological interactions analysis. Throughout, our multiple-comparison correction alpha always remained at or below conventional p values.
The differences in heart rate were not included in the fMRI analyses since previous studies have shown that changes in heart rate do not have a significant effect on global blood flow (16, 17).
Functional connectivity analyses
Functional connectivity analyses are able to detect regions whose BOLD response activity covaries with the activity of a selected reference voxel in a brain region of interest. Regions that exhibit significant covariance with the activity of the reference voxel over the time course of the task being studied, but not during the baseline condition scans, are inferred to be functionally connected to the region represented by the reference voxel (18). The approach of choosing a seed voxel and correlating the time series of the seed voxel (or cluster of seed voxels) is one of many approaches used to determine functional connectivity. These approaches range from nondirectional multivariate analyses (e.g., principal-component analysis and certain versions of canonical correlation [19]) to path analytic approaches (20). Like path analysis, the present method is region selective; however, like exploratory methods, it is mute in reference to the predominant direction of interregion activation.
To determine which voxel’s time series data would be used in the psychophysiological interaction analyses, we conducted a large subtraction analysis in which all subjects’ data were analyzed together to determine areas of commonly significant BOLD response elicited by performance of the script-driven imagery task relative to the baseline BOLD response. The subtraction analysis in which all subjects’ baseline scans were averaged and subtracted from all subjects’ averaged activation scans yielded maximally activated voxels in the right anterior cingulate at coordinates x=2, y=20, z=36; the left middle frontal at coordinates x=–28; y=48, z=–8; and the left posterior cingulate gyrus at coordinates x=–1, y=–20, z=42 (SPM[t], p<0.0001, corrected for multiple comparisons; coordinates in standardized stereotaxic space of Talairach and Tournoux [21]). For each subject, BOLD response time series values were extracted from these voxels. After data extraction, each subject’s vector of voxel activity values was normed, yielding 24 vectors whose elements had a mean of zero and a standard deviation of 1. Each normed vector was multiplied in a point-wise fashion by the hemodynamically convolved boxcar-shaped vector that was originally used in the subtraction analysis to estimate the effects attributable to the traumatic script-driven imagery transaction. Through this procedure, the measurement period that is registered is temporally offset to accommodate the hemodynamically induced lag in the BOLD response expression of target activation. One hemodynamically convolved boxcar-shaped vector was produced per subject. These vectors were entered into one of two group SPM analyses as covariates of interest to produce statistical parametric maps of the functional connectivity for the right anterior cingulate gyrus, left posterior cingulate gyrus, and left middle frontal gyrus for the traumatized subjects with and without PTSD. For each subject group, linear contrasts were utilized to display the location and extent of the brain regions whose activity demonstrated a significant psychophysiological interaction with the right anterior cingulate gyrus, left posterior cingulate gyrus, or left middle frontal gyrus in the form of SPM(t) maps (corrected for multiple comparisons, thresholded at p<0.05).
Results
All PTSD patients experienced the traumatic memories in the form of flashbacks. They reported feeling like “I was back at the scene of the accident” or “It felt like I was back in the past.” All comparison subjects recalled the traumatic event as an ordinary autobiographical memory.
The PTSD patients showed a significantly greater increase from baseline in heart rate during the script-driven imagery condition, relative to the comparison subjects (increase in PTSD group: mean=13.51 bpm, SD=6.96; increase in comparison group: mean=1.08 bpm, SD=3.62) (t=–5.34, df=14.4, p<0.00001, one-tailed).
Brain Activation During Script-Driven Imagery
Subtraction analyses
Table 1 shows regions of activation during traumatic memory recall in the comparison (N=13) and PTSD groups (N=11). Both groups showed activation in the middle frontal gyrus and various regions of the cingulate gyrus. The time courses of activation showed that brain activation returned to baseline during rest periods in all brain areas studied for both the PTSD group and the comparison group. These time courses indicated that 60 seconds was enough time for subjects to recover from the traumatic script-driven remembrances. Baseline brain activation did not differ significantly between the PTSD patients and the comparison subjects (data not shown).
Recalling a neutral memory resulted in the activation of the right anterior cingulate gyrus (Brodmann’s area 32), right middle and inferior frontal gyri (Brodmann’s area 10), left parietal lobe (Brodmann’s areas 7 and 40), and occipital lobe (Brodmann’s area 18) in both the PTSD patients and the comparison subjects. Compared with the PTSD subjects, the comparison subjects showed significantly (p<0.001) more activation in only one cluster (parietal lobe [Brodmann’s area 7]) during recall of a neutral memory. Relative to the comparison subjects, the PTSD subjects showed significantly (p<0.001) more activation in the superior frontal gyrus (Brodmann’s area 10) and thalamus during recall of a neutral memory (data not shown).
Functional connectivity analyses
Comparison of the two study groups’ SPM(t) maps examining the psychophysiological interaction between activity in the right anterior cingulate cortex (coordinates x=2, y=20, z=36) and traumatic script-driven imagery (Table 2) showed that the comparison subjects had more significant correlation than the PTSD subjects in the left superior frontal gyrus (Brodmann’s area 9), left anterior cingulate gyrus (Brodmann’s area 32), left striatum (caudate), left parietal lobe (Brodmann’s areas 40 and 43), and left insula (Brodmann’s area 13) (Figure 1). In contrast, the PTSD subjects had more significant correlation than the comparison subjects in the right posterior cingulate gyrus (Brodmann’s area 29), right caudate, right parietal lobe (Brodmann’s areas 7 and 40), right occipital lobe (Brodmann’s area 19), right inferior frontal gyrus (Brodmann’s area 9), right middle temporal gyrus (Brodmann’s areas 19 and 20), left insula (Brodmann’s areas 13 and 47), and left thalamus (Figure 2).
Functional connectivity analyses were attempted with common regions of activation other than the right anterior cingulate cortex (Brodmann’s area 32), namely the left middle frontal gyrus (coordinates x=–28, y=48, z=–8) and the left posterior cingulate gyrus (coordinates x=–1, y=–20, z=42). Comparison of the two study groups’ SPM(t) maps examining psychophysiological interactions between the activity in the left middle frontal gyrus (coordinates x=–28, y=48, z=–8) and traumatic script-driven imagery showed that the PTSD subjects had greater correlations than the comparison subjects in the right middle frontal gyrus (coordinates x=44, y=16, z=34) (p<0.0001, t=6.67, df=1680).
Comparison of the two study groups’ SPM(t) maps examining psychophysiological interactions between activity in the left posterior cingulate gyrus (coordinates x=–1, y=–20, z=42) activity and traumatic script-driven imagery showed that the comparison subjects had greater correlation than the PTSD subjects in the left insula (coordinates x=–34, y=22, z=10) (t=8.86, df=1680, p<0.0001), left thalamus (coordinates x=–6,y=–22, z=4) (t=8.58, df=1680, p<0.0001), left medial frontal gyrus (coordinates x=–12, y=62, z=–6; x=–6, y=60, z=22; and x=–8, y=46, z=4) (t=8.03, df=1680, p<0.0001), right medial frontal gyrus (coordinates x=4, y=42, z=28) (t=7.57, df=1680, p<0.0001), and right cingulate gyrus (coordinates x=6, y=16, z=26; x=6, y=–2, z=32) (t=7.51, df=1680, p<0.0001). In contrast, the PTSD subjects had greater correlation than the comparison subjects in the left insula (coordinates x=–42, y=14, z=0) (t=8.89, df=1680, p<0.0001), left precuneus (coordinates x=–12, y=–56, z=50) (t=8.13, df=1680, p<0.0001), right anterior cingulate gyrus (coordinates x=4, y=16, z=–6) (t=7.83, df=1680, p<0.0001), and right subcallosal gyrus (coordinates x=14, y=16, z=–10; x=10, y=2, z=–12) (t=6.86, df=1680, p<0.0001).
Discussion
The comparison of functional connectivity maps showed distinctly different connectivity patterns in the PTSD subjects and in the comparison subjects. The most striking findings arose from the connectivity analyses originating from activation in the cognitive division of the right anterior cingulate gyrus (Brodmann’s area 32) (coordinates x=2, y=20, z=36). The affective division of the anterior cingulate gyrus has been shown to be involved during the recall of traumatic material in PTSD (2–4, 7), and reciprocal connections between the cognitive and affective divisions of the anterior cingulate gyrus have been described (22). In addition, the affective division of the anterior cingulate gyrus plays a role in the conscious experience of emotion and in linking autonomic changes to emotional stimuli (22, 23). PTSD subjects did not show similar patterns of alterations in brain activation during recall of a neutral autobiographical memory. It is therefore unlikely that the between-group differences observed in response to the traumatic memories are generalizable to any autobiographical memory.
The differences in functional connectivity patterns observed between the PTSD subjects and the comparison subjects may be related to variations in episodic memory retrieval. All PTSD patients experienced the traumatic memories in the form of flashbacks, whereas the comparison subjects recalled the traumatic events as ordinary autobiographical memories.
Reexperiencing of traumatic events in the form of flashbacks is very different from the recall of events as ordinary autobiographical memories (24, 25). Flashbacks often occur spontaneously and are triggered by internal or external events, and their occurrence usually cannot be controlled. Flashbacks also involve a subjective distortion in time. They are much more vivid in nature and are often experienced as though the event were happening again in the present. Flashbacks have also been shown to be experienced as fragments of the sensory components of the event, such as visual images or olfactory, auditory, or kinesthetic sensations (25). They have also been reported to be unchanging over time (26), compared with ordinary memories, which are altered by repeated recall (27). Overall, flashbacks are therefore highly perceptual memories that are primarily image-based. In contrast, in ordinary autobiographical memory, the sensory elements of the experience are automatically integrated into a personal narrative (26).
It is interesting to note that the functional connectivity analyses for the comparison subjects showed areas of brain activation that are consistent with verbal episodic memory retrieval. Comparison of the functional connectivity maps of the PTSD subjects and the comparison subjects showed that the comparison subjects had greater levels of brain activation in the left superior frontal gyrus (Brodmann’s area 9), left anterior cingulate gyrus (Brodmann’s area 32), left striatum (caudate), left parietal lobe (Brodmann’s areas 40 and 43), and left insula (Brodmann’s area 13). Prefrontal activations during episodic memory retrieval have sometimes been shown to be bilateral, but overall to have a clear tendency for right lateralization (28). Anterior cingulate activation during memory recall has been hypothesized to be related to language processes, since activation of this area is more frequent for verbal than nonverbal materials.
The functional connectivity analyses for PTSD patients revealed a much more nonverbal pattern of memory retrieval. Comparison of the functional connectivity maps of the PTSD subjects and the comparison subjects showed that the PTSD subjects had greater levels of brain activation in the right posterior cingulate gyrus (Brodmann’s area 29), right caudate, right parietal lobe (Brodmann’s areas 7 and 40), and right occipital lobe (Brodmann’s area 19). For nonverbal episodic retrieval, occipital, right parietal, and posterior cingulate activations have been shown to predominate (28, 29). Moreover, the posterior cingulate gyrus has recently been shown to mediate interactions of emotional and memory-related processes (30). Functional connectivity analyses may therefore help to elucidate the differences in the nature of memory recall (verbal versus nonverbal) between comparison subjects and PTSD patients. It is interesting to note that Paivio (31) has proposed a dual coding theory in which affective and emotional reactions become associated primarily with a nonverbal representational system because they are learned in the context of nonverbal events.
Functional neuroimaging techniques (PET and fMRI) have been used to investigate the neuronal circuitry underlying autobiographical memory. In a review of 11 neuroimaging studies of autobiographical memory, Maguire (32) noted that the most consistent result seen is a medial and left-lateralized activation pattern (33, 34). In one study (35), however, subjects were instructed to recall particularly affect-laden memories, resulting in a predominantly right hemispheric activation pattern that included the temporal cortex, posterior cingulate, insula, and prefrontal cortex. Our results add an interesting dimension to this literature, as they suggest that the subjects without PTSD do not process their “traumatic” memories as affect-laden memories, whereas PTSD subjects show the right-lateralized pattern of activation consistent with the results of Fink et al. (33).
The functional connectivity analyses also found differences in the brain regions whose activity covaried with that of the left posterior cingulate gyrus. Of particular interest was the finding that activity in the right cingulate gyrus was lower in the PTSD subjects than in the comparison subjects, while activity in the right anterior cingulate gyrus was higher in the PTSD subjects than in the comparison subjects. There are reciprocal connections between the rostral cingulate and both the dorsal anterior and subgenual cingulate gyrus (36). Mayberg et al. (37, 38) found that pretreatment metabolism in the rostral anterior cingulate gyrus uniquely differentiated treatment responders from nonresponders in a group of patients with unipolar depression. Furthermore, activity in both the left posterior cingulate (Brodmann’s area 31) and right dorsal anterior cingulate (Brodmann’s area 24b) increased after 6 weeks of treatment with fluoxetine in the treatment responders. In studies of patients with obsessive-compulsive disorder (OCD), Rauch et al. found that high pretreatment activation levels in the posterior cingulate cortex predicted treatment response to fluvoxamine (39) and to anterior cingulotomy (40). These predictive findings may be relevant to our study for two reasons. First, there is a high degree of comorbidity in patients with PTSD, who often experience depression and/or other anxiety disorders, raising the question of whether similar predictive patterns may be seen in PTSD patients treated with these agents. Second, given these similarities in activation pattern between the anxiety disorders and depression, follow-up studies will be necessary to examine whether consistent differences in activation patterns might serve to differentiate between the two groups of patients.
Functional connectivity analyses also suggested differences in laterality, with PTSD subjects showing a right hemisphere dominance effect. The importance of lateralized responses in PTSD has been previously examined by using EEG and auditory probe evoked potential attenuation. Schiffer et al. (41), using auditory probe evoked potential attenuation as a measure of hemispheric activity, reported that subjects who had experienced early trauma displayed significant left-dominant asymmetry during neutral memory recall and relative right dominance during traumatic memory recall. In addition, psychological abuse has been shown to be associated with an increased prevalence of left-sided EEG abnormalities and an increased prevalence of right-left hemisphere asymmetries (42). EEG coherence studies also reported that abused children had greater average left hemisphere coherence than did nonabused children but that the two groups had a comparable degree of right hemisphere coherence. Teicher et al. (43) suggested that these findings may be related to diminished left hemisphere differentiation in the abused group and thus may provide evidence that childhood abuse has a significant effect on cortical development.
Previous neuroimaging studies in PTSD using the script-driven imagery symptom provocation paradigm have also suggested lateralized responses. Using traumatic script-driven imagery, Rauch et al. (8) found that in the traumatic condition, relative to the neutral condition, regional cerebral blood flow increases occurred in the right medial orbitofrontal cortex, insula, amygdala, and anterior temporal pole. However, lateralized responses have been reported in other disorders besides PTSD. Pooling data from patients with three different anxiety disorders, including OCD, simple phobia, and PTSD in a study that used symptom provocation paradigms, Rauch et al. (44) found that the right inferior and medial orbital prefrontal cortex as well as the bilateral insular cortex, lenticulate nuclei, and brainstem foci were consistently activated across the three different groups. These provocation studies indicate the consistent involvement of both paralimbic and subcortical regions in the mediation of acute anxiety, independent of stimulus type and patient group. These results illustrate that the specific mechanisms underlying the different anxiety disorders remain unclear and that studies are needed to address functional connectivity/neuronal networks rather than solely examining specific brain regions involved in the acute anxiety experience. The importance of examining neuronal networks in addition to regionally specific changes has also recently been described by Shaw et al. (45), who used covariance-based multivariate analysis to investigate distributed brain systems in PTSD patients and matched comparison subjects during performance of a working memory task. However, it is not possible to directly compare our findings to those of Shaw et al. because of the different nature of the memory systems (autobiographical memory versus working memory) investigated in the two studies.
The present study had several limitations that must be considered in interpreting the findings. The relatively small number of study participants (N=11 in the PTSD group and N=13 in the comparison group) was not large enough for a random-effects analysis, which would have allowed the extrapolation of the results from this study to a larger population. Studies using a larger number of subjects are currently in progress. In addition, the comorbidity profile of the PTSD subjects may be a confounding factor in the study. Some PTSD subjects included in the present study had significant comorbidity, including dysthymic disorder, panic disorder, lifetime history of polysubstance dependence, and major depressive disorder. Furthermore, the current study included subjects who had experienced a mixture of traumata (sexual/physical abuse and motor vehicle accidents). Future studies will need to address possible pretrauma differences in imagery and verbal thinking habits between PTSD subjects and comparison subjects.
In summary, the present functional connectivity findings illustrate significant differences in brain connectivity between PTSD and comparison subjects during the recall of traumatic events. These variations may account for the differences in the nature (i.e., nonverbal versus verbal) of traumatic memory recall between PTSD subjects and comparison subjects. Moreover, the connectivity analyses used in this study may also allow a better understanding of the functional connectivity underlying various anxiety disorders and depression. Many of the same patterns of activation are seen in paralimbic and subcortical structures in both types of disorders, and there is a high degree of comorbidity between the two types. Functional connectivity analyses may help to elucidate the differences in the dynamic neuronal networks involved in these disorders.
![]() |
![]() |
Received Dec. 16, 2002; revision received May 22, 2003; accepted May 29, 2003. From the Departments of Psychiatry, Psychology, and Medical Biophysics, University of Western Ontario, London, Ontario, Canada. Address reprint requests to Dr. Lanius, Department of Psychiatry, London Health Sciences Centre, 339 Windermere Rd., P.O. Box 5339, London, Ont., Canada N6A 5A5; [email protected] (e-mail). Supported by grants from the Canadian Institutes of Health Research and the Ontario Mental Health Foundation. The authors thank Drs. Al Paivio, James Hopper, and Robyn Bluhm for helpful comments on the manuscript.
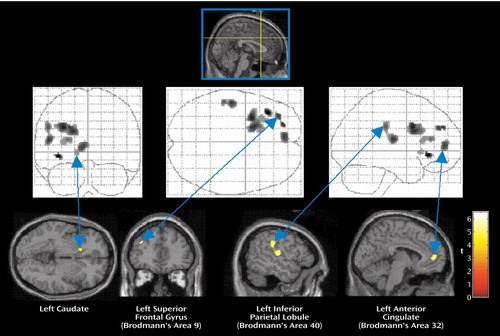
Figure 1. Brain Regions With Activation Showing Significantly Greater Connectivity/Covariation With Activation in the Right Anterior Cingulate in Traumatized Subjects Without PTSD Than in Traumatized Subjects With PTSD During Recall of a Traumatic Eventa
aAreas of connectivity/covariation determined by the statistical parametric map of the t statistic (SPM[t]) showing the psychophysiological interaction between activity in the right anterior cingulate cortex (Talairach coordinates x=2, y=20, z=36) and activity in other brain regions. The grid diagrams show all areas with significantly greater covariation in the subjects without PTSD. The cross-sectional brain images show sites of significant covariation in areas of interest.
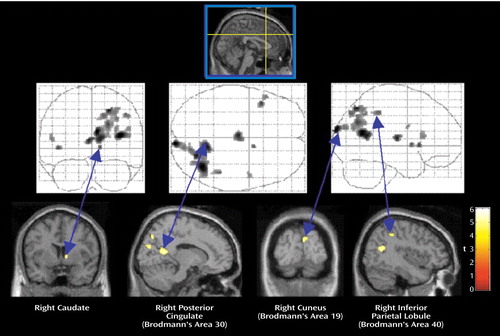
Figure 2. Brain Regions With Activation Showing Significantly Greater Connectivity/Covariation With Activation in the Right Anterior Cingulate in Traumatized Subjects With PTSD Than in Traumatized Subjects Without PTSD During Recall of a Traumatic Eventa
aAreas of connectivity/covariation determined by the statistical parametric map of the t statistic (SPM[t]) showing the psychophysiological interaction between activity in the right anterior cingulate cortex (Talairach coordinates x=2, y=20, z=36) and activity in other brain regions. The grid diagrams show all areas with significantly greater covariation in the subjects with PTSD. The cross-sectional brain images show sites of significant covariation in areas of interest.
1. Osuch EA, Benson B, Geraci M, Podell D, Herscovitch P, McCann UD, Post RM: Regional cerebral blood flow correlated with flashback intensity in patients with posttraumatic stress disorder. Biol Psychiatry 2001; 50:246–253Crossref, Medline, Google Scholar
2. Bremner JD, Narayan M, Staib LH, Southwick SM, McGlashan T, Charney DS: Neural correlates of memories of childhood sexual abuse in women with and without posttraumatic stress disorder. Am J Psychiatry 1999; 156:1787–1795Abstract, Google Scholar
3. Bremner JD, Staib L, Kaloupek D, Southwick SM, Soufer R, Charney DS: Neural correlates of exposure to traumatic pictures and sound in Vietnam combat veterans with and without posttraumatic stress disorder: a positron emission tomography study. Biol Psychiatry 1999; 45:806–816Crossref, Medline, Google Scholar
4. Liberzon I, Taylor SF, Amdur R, Jung TD, Chamberlain KR, Minoshima S, Koeppe RA, Fig LM: Brain activation in PTSD in response to trauma-related stimuli. Biol Psychiatry 1999; 45:817–826Crossref, Medline, Google Scholar
5. Shin LM, Kosslyn SM, McNally RJ, Alpert NM, Thompson WL, Rauch SL, Macklin ML, Pitman RK: Visual imagery and perception in posttraumatic stress disorder: a positron emission tomographic investigation. Arch Gen Psychiatry 1997; 54:233–241Crossref, Medline, Google Scholar
6. Shin LM, McNally RJ, Kosslyn SM, Thompson WL, Rauch SL, Alpert NM, Metzger LJ, Lasko NB, Orr SP, Pitman RK: Regional cerebral blood flow during script-driven imagery in childhood sexual abuse-related PTSD: a PET investigation. Am J Psychiatry 1999; 156:575–584Abstract, Google Scholar
7. Lanius RA, Williamson PC, Densmore M, Boksman K, Gupta MA, Neufeld RW, Gati JS, Menon RS: Neural correlates of traumatic memories in posttraumatic stress disorder: a functional MRI investigation. Am J Psychiatry 2001; 158:1920–1922Link, Google Scholar
8. Rauch SL, van der Kolk BA, Fisler RE, Alpert NM, Orr SP, Savage CR, Fischman AJ, Jenike MA, Pitman RA: A symptom provocation study of posttraumatic stress disorder using positron emission tomography and script driven imagery. Arch Gen Psychiatry 1996; 53:380–387Crossref, Medline, Google Scholar
9. Hull AM: Neuroimaging findings in post-traumatic stress disorder: systematic review. Br J Psychiatry 2002; 181:102–110Crossref, Medline, Google Scholar
10. Bremner JD: Neuroimaging studies in post-traumatic stress disorder. Curr Psychiatry Rep 2002; 4:254–263Crossref, Medline, Google Scholar
11. Shin LM, Whalen PJ, Pitman RK, Bush G, Macklin ML, Lasko NB, Orr SP, McInerney SC, Rauch SL: An fMRI study of anterior cingulate function in posttraumatic stress disorder. Biol Psychiatry 2001; 50:932–942Crossref, Medline, Google Scholar
12. Blake DD, Weathers FW, Nagy LM, Kaloupek DG, Gusman FD, Charney DS, Keane TM: The development of a Clinician-Administered PTSD Scale. J Trauma Stress 1995; 8:75–90Crossref, Medline, Google Scholar
13. Lanius RA, Williamson PC, Boksman K, Densmore M, Gupta M, Neufeld RWJ, Gati JS, Menon RS: Brain activation during script-driven imagery induced dissociative responses in PTSD: a functional magnetic resonance imaging investigation. Biol Psychiatry 2002; 52:305–311Crossref, Medline, Google Scholar
14. Lanius RA, Williamson PC, Hopper J, Densmore M, Boksman K, Gupta MA, Neufeld, RWJ, Gati JS, Menon RS: Recall of emotional states in posttraumatic stress disorder: a functional MRI investigation. Biol Psychiatry 2003; 53:204–210Crossref, Medline, Google Scholar
15. Worsley KJ, Friston KJ: Analysis of fMRI time-series revisited—again. Neuroimage 1995; 2:173–181Crossref, Medline, Google Scholar
16. King AB, Menon RS, Hachinski V, Cechetto DF: Human forebrain activation by visceral stimuli. J Comp Neurol 1999; 413:572–582Crossref, Medline, Google Scholar
17. Ploghaus A, Tracey I, Gati JS, Clare S, Menon RS, Matthews PM, Rawlins JN: Dissociating pain from its anticipation in the human brain. Science 1999; 284:1979–1981Crossref, Medline, Google Scholar
18. Friston KJ, Frith CD, Liddle PF, Frackowiak RSJ: Functional connectivity: the principal-component analysis of large (PET) data sets. J Cereb Blood Flow Metab 1993; 13:5–14Crossref, Medline, Google Scholar
19. Shaw ME, Strother SC, McFarlane AC, Morris P, Anderson J, Clark CR, Egan GF: Abnormal functional connectivity in posttraumatic stress disorder. Neuroimage 2002; 15:661–674Crossref, Medline, Google Scholar
20. Neufeld RWJ: Clinical Quantitative Methods. New York, Grune & Stratton, 1977Google Scholar
21. Talairach J, Tournoux P: Co-Planar Stereotaxic Atlas of the Human Brain: Three-Dimensional Proportional System. Stuttgart, Germany, Georg Thieme, 1988Google Scholar
22. Bush G, Luu P, Posner MI: Cognitive and emotional influences in anterior cingulate cortex. Trends Cogn Sci 2000; 4:215–222Crossref, Medline, Google Scholar
23. Lane RD, Reiman EM, Ahern GL, Schwartz GE, Davidson RJ: Neuroanatomical correlates of happiness, sadness, and disgust. Am J Psychiatry 1997; 154:926–933Link, Google Scholar
24. Brewin CR, Dalgleish T, Joseph S: A dual representation theory of post traumatic stress disorder. Psychol Rev 1996; 103:670–686Crossref, Medline, Google Scholar
25. Van der Kolk BA, Fisler R: Dissociation and the fragmentary nature of traumatic memories: overview and exploratory study. J Trauma Stress 1995; 8:505–525Crossref, Medline, Google Scholar
26. Van der Kolk BA: Trauma and memory, in Traumatic Stress: The Effects of Overwhelming Experience on Mind, Body, and Society. Edited by van der Kolk BA, McFarlane AC, Weisaeth L. New York, Guilford, 1996, pp 279–302Google Scholar
27. Estes WK: Processes of memory loss, recovery, and distortion. Psychol Rev 1997; 104:148–169Crossref, Medline, Google Scholar
28. Cabeza R, Nyberg L: Imaging cognition, II: an empirical review of 275 PET and fMRI studies. J Cogn Neurosci 2000; 12:1–47Crossref, Medline, Google Scholar
29. Cabeza R, Nyberg L: Imaging cognition: an empirical review of PET studies with normal subjects. J Cogn Neurosci 1997; 9:1–26Crossref, Medline, Google Scholar
30. Maddock RJ, Garrett AS, Buonocore MH: Posterior cingulate cortex activation by emotional words: fMRI evidence from a valence decision task. Hum Brain Mapp 2003; 18:30–41Crossref, Medline, Google Scholar
31. Paivio A: Mental Representations: A Dual Coding Approach. New York, Oxford University Press, 1986, p 271Google Scholar
32. Maguire EA: Neuroimaging studies of autobiographical event memory. Phil Trans R Soc Lond B 2001; 336:1441–1451Crossref, Google Scholar
33. Fink GR, Markowitsch HJ, Reinkemeier M, Bruckbauer T, Kessler J, Heiss W-D: Cerebral representation of one’s own past: neural networks involved in autobiographical memory. J Neurosci 1996; 16:4275–4282Crossref, Medline, Google Scholar
34. Conway MA, Turk DJ, Miller SL, Logan J, Nebes RD, Meltz CC, Becker JT: A positron emission tomography (PET) study of autobiographical memory retrieval. Memory 1999; 7:679–702Crossref, Medline, Google Scholar
35. Maddock RJ, Garrett AS, Buonocore MH: Remembering familiar people: the posterior cingulate cortex and autobiographical memory retrieval. Neuroscience 2001; 104:667–676Crossref, Medline, Google Scholar
36. Vogt BA, Nimchinsky EA, Vogt LJ, Hof PR: Human cingulate cortex: surface features, flat maps, and cytoarchitecture. J Comp Neurol 1995; 359:490–506Crossref, Medline, Google Scholar
37. Mayberg HS, Brannan SK, Mahurin RK, Jerabek PA, Brickman JS, Tekell JL, Silva JA, McGinnis S, Glass TG, Martin CC, Fox PT: Cingulate function in depression: a potential predictor of treatment response. Neuroreport 1997; 8:1057–1061Crossref, Medline, Google Scholar
38. Mayberg HS, Brannan SK, Tekell JL, Silva JA, Mahurin RK, McGinnis S, Jerabek PA: Regional metabolic effects of fluoxetine in major depression: serial changes and relationship to clinical response. Biol Psychiatry 2000; 48:830–843Crossref, Medline, Google Scholar
39. Rauch SL, Shin LM, Dougherty DD, Alpert NM, Fischman AJ, Jenike MA: Predictors of fluvoxamine response in contamination-related obsessive-compulsive disorder: a PET symptom provocation study. Neuropsychopharmacology 2002; 27:782–791Crossref, Medline, Google Scholar
40. Rauch SL, Dougherty DD, Cosgrove GR, Cassem EH, Alpert NM, Price BH, Nierenberg AA, Mayberg HS, Baer L, Jenike MA, Fischman AJ: Cerebral metabolic correlates as potential predictors of response to anterior cingulotomy for obsessive compulsive disorder. Biol Psychiatry 2001; 50:659–667Crossref, Medline, Google Scholar
41. Schiffer F, Teicher MH, Papanicolaou AC: Evoked potential evidence for right brain activity during recall of traumatic memories. J Neuropsychiatry Clin Neurosci 1995; 7:169–175Crossref, Medline, Google Scholar
42. Ito Y, Teicher MH, Glod CA, Harper D, Magnus E, Gelbard HA: Increased prevalence of electrophysiological abnormalities in children with psychological, physical, and sexual abuse. J Neuropsychiatry Clin Neurosci 1993; 5:401–408Crossref, Medline, Google Scholar
43. Teicher MH, Ito Y, Glod CA, Andersen SL, Dumont N, Ackerman E: Preliminary evidence for abnormal cortical development in physically and sexually abused children using EEG coherence and MRI. Ann NY Acad Sci 1997; 821:160–175Crossref, Medline, Google Scholar
44. Rauch SL, Savage CR, Alpert NM, Fischman AJ, Jenike MA: The functional neuroanatomy of anxiety: a study of three disorders using positron emission tomography and symptom provocation. Biol Psychiatry 1997; 42:446–452Crossref, Medline, Google Scholar
45. Shaw ME, Strother SC, McFarlane AC, Morris P, Anderson J, Clark CR, Egan GF: Abnormal functional connectivity in posttraumatic stress disorder. Neuroimage 2002; 15:661–674Crossref, Medline, Google Scholar