Decreased Striatal Dopamine D1 Receptor- Stimulated Adenylyl Cyclase Activity in Human Methamphetamine Users
Abstract
OBJECTIVE: It has been assumed that some behavioral changes associated with repeated exposure to dopaminergic psychostimulant drugs might be explained by changes in activity of dopamine receptors, including the dopamine D1 receptor, which is linked by a stimulatory G protein to the effector enzyme adenylyl cyclase. To establish whether dopamine D1 receptor function might be altered in human methamphetamine users, the authors measured dopamine-stimulated adenylyl cyclase activity in the brain of chronic human users of the drug. METHOD: Adenylyl cyclase activity stimulated by dopamine and by guanylyl-imidodiphosphate (to assess G protein and adenylyl cyclase coupling) was determined in the postmortem brain tissue of 16 methamphetamine users who had used the drug both recently and chronically (i.e., at least 1 year) as well as 21 matched comparison subjects. RESULTS: A 25%–30% decrease in the maximal extent of dopamine stimulation of adenylyl cyclase activity was seen in the striatum (nucleus accumbens, caudate, and putamen) of the methamphetamine users. No changes were found in basal or guanylyl-imidodiphosphate-stimulated enzyme activity. CONCLUSIONS: These data suggest that dopamine receptor function linked to adenylyl cyclase is partially desensitized in the striatum of human methamphetamine users. Decreased dopamine D1 receptor function might underlie part of the known (drug withdrawal syndrome) or expected (drug tolerance) consequences of methamphetamine exposure in humans.
Methamphetamine is a highly addictive psychostimulant that is widely abused for the euphoric effects of the drug. The chronic effects of methamphetamine exposure in some, but not all, regular drug users include an extended drug withdrawal syndrome that can be characterized by separate periods of dysphoria and drug craving (1). It is also assumed that with repeated use of methamphetamine, some tolerance usually occurs to the pleasurable effects of the drug (2). It is surprising, however, that the question of acute or chronic tolerance has not yet been rigorously established in chronic human users of the drug (3).
Methamphetamine shares with other drugs of abuse (e.g., cocaine, heroin, alcohol) the ability to enhance release of the neurotransmitter dopamine in the striatum (caudate, putamen, nucleus accumbens) (4, 5), with the extent of the dopamine increase correlated with the intensity of the “high” in humans (6, 7). For this reason, it continues to be assumed that some of the effects of methamphetamine exposure in humans are explained in large part by alterations in activity of the pre- and postsynaptic dopamine system in the brain (8). Thus, we previously reported that striatal tissue levels of dopamine can be low in some users of methamphetamine (9)—as low as those in Parkinson’s disease in some striatal subdivisions (10)—which suggests that some of the unpleasant consequences of methamphetamine during drug withdrawal (e.g., depression, cognitive impairment) might be due in part to a striatal dopamine deficiency. It is also possible that short- and long-term adaptive changes due to overactivation of dopamine receptors occurs in methamphetamine-exposed human brain, which might explain some aspects of drug-taking behavior.
Dopamine receptor types can be classified by their ability to stimulate (D1 receptors) or inhibit (D2 receptors) adenylyl cyclase (EC 4.6.1.1) through the mediation of the stimulatory G protein (Gs or, in striatum, Golf[11]) or the inhibitory G protein (Gi, Go), respectively (see reference 12 for a review). Since the pharmacological literature on the influence of dopamine D1 receptor agonists/antagonists on the behavior of human psychostimulant users is scanty, the role of the dopamine D1 receptor in psychostimulant use and abuse in the human has not yet been established. However, the preliminary observation that a single dose of a dopamine D1 receptor antagonist (SCH 39166, ecopipam) can partially block the euphoric effects of cocaine in chronic users of the psychostimulant (13) suggests that the dopamine D1 receptor might mediate part of the pleasurable effects of dopaminergic stimulants. Although no data have yet been provided indicating that dopamine D1 receptor agonists are rewarding to humans (14), agonists acting on this receptor are self-administered by nonhuman primates (15, 16).
Apart from these pharmacological studies, information on the status of the dopamine D1 receptor system in human psychostimulant users is limited to our postmortem finding of normal levels of dopamine D1 receptor protein in the dopamine-rich striatal subdivisions of methamphetamine users and users of cocaine, with the exception of higher protein concentration of the receptor restricted to the nucleus accumbens subdivision in the methamphetamine users (17). Changes or lack of changes in brain neurotransmitter receptor concentrations can, at most, only suggest differences in receptor function. Therefore, we have now extended our investigation to include measurement, in the postmortem brain tissue of chronic methamphetamine users, of a more dynamic index of dopamine D1 receptor function, namely dopamine-stimulated adenylyl cyclase activity, since adenylyl cyclase is considered to be the key effector of dopamine D1 receptor function (12). In order to establish the possible site and specificity of any disturbance in dopamine D1 receptor, G protein, and adenylyl cyclase coupling, we also measured stimulation of adenylyl cyclase by a nonhydrolyzable GTP analog guanylyl-imidodiphosphate [Gpp(NH)p], which stimulates adenylyl cyclase by direct activation of the stimulatory G protein, i.e., bypassing the dopamine receptor, thereby assessing the G protein and adenylyl cyclase coupling. We report that dopamine D1-stimulated adenylyl cyclase activity is decreased in striatum of human methamphetamine users, a finding that might explain some of the short- or long-term aspects of drug-taking behavior.
Method
Brain Materials
Brains from a total of 21 comparison subjects (19 men and two women) and 16 chronic users of methamphetamine (11 men and five women) were obtained postmortem from medical examiner offices in the United States per a standardized protocol. Informed consent was obtained from the next of kin. The comparison subjects and methamphetamine users did not significantly differ in age (mean=33.6 years [SD=10.1] and 32.4 years [SD=8.1], respectively; t=0.38, df=35, p=0.70) or postmortem interval between death and freezing of the brain (mean=13.1 hours [SD=5.8] and 15.3 hours [SD=6.7]; t=1.1, df=35, p=0.29). At autopsy, half of the brain was fixed in formalin fixative for neuropathological analysis, whereas the other half was immediately frozen until dissection for neurochemical analysis. Samples of femoral blood were obtained from all of the methamphetamine users and the comparison subjects for drug screening. Scalp hair samples for drug analyses were available for 18 of the 21 comparison subjects and 12 of the 16 methamphetamine users. Levels of drugs of abuse in blood and other bodily fluids were measured by the local medical examiner; drug analyses in brain and hair samples were conducted by one of the investigtors (K.K.) at the Armed Forces Institute of Pathology in Washington, D.C. All methamphetamine users met the following selection criteria: 1) presence of methamphetamine confirmed by toxicology screening analyses of blood, autopsied brain, and, when available, sequential scalp hair samples; 2) absence of any other drugs of abuse (including blood ethanol) in these tissues; 3) evidence of methamphetamine use for at least 1 year before death (obtained from case records and structured interviews with medical examiner investigators, next of kin, and informants); and 4) absence of neurological illness or brain pathology unrelated to use of the drug. Drug histories and patient information are summarized in Table 1, with information on 12 of the 16 methamphetamine users previously reported (9). The regional distribution of methamphetamine and its metabolite amphetamine in autopsied brain has been reported for 12 of the 16 methamphetamine subjects (18).
All comparison subjects were neurologically normal, had no evidence of brain pathology, had no history of drug use, and tested negative for all drugs of abuse in blood, autopsied brain, and, in the 18 subjects from whom it was available, sequential scalp hair samples. The causes of death for the comparison subjects were cardiovascular disease (N=12), trauma (N=7), drowning (N=1), and leukemia (N=1).
Adenylyl Cyclase Assay
Homogenates, in 50 mM Tris-HCl, pH 7.4, 0.1 mM CaCl2, 1% (vol/vol) protease inhibitors (Sigma, catalog number P8340), of dissected brain samples (nucleus accumbens, caudate, putamen, frontal cortex [Brodmann’s area 9], and temporal cortex [Brodmann’s area 22]) were used. The procedure for the assay of dopamine-stimulated adenylyl cyclase activity was the same as reported previously (19) with minor modifications. Enzyme activity in brain homogenates (20 μg protein) was assayed in a total volume of 100 μl containing 50 mM Tris-HCl buffer, pH 7.4, 0.5 mM MgCl2, 0.2 mM EGTA, 4 mM phosphocreatine, 25 units/ml of creatine phosphokinase, 1% (vol/vol) protease inhibitors, 1 mM cAMP, 10 μM GTP, 0.2 mM ATP, and 1 μCi [α-33P]ATP in the absence and presence of varying concentrations (0.4–400 μM, seven concentrations) of dopamine. In the present study, the concentration of Mg++ was lowered to 0.5 mM (versus 4 mM in reference 19) in the assay, since this modification produced lower basal adenylyl cyclase activity and therefore higher percentage dopamine stimulation in the striatal tissues (30%–40% versus approximately 20%). The percentage stimulation in the cerebral cortex was not changed by the modification.
For the assay of Gpp(NH)p stimulation, GTP was replaced with varying concentrations (10–8 to 10– 4 M, five concentrations) of Gpp(NH)p, and the concentration for MgCl2 was 5 mM. Under the conditions used, i.e., high ATP concentration, high temperature (30°C), and without an additional factor (e.g., forskolin, calcium/calmodulin) to elevate basal adenylyl cyclase activity, Gpp(NH)p preferentially activates Gs/Golf rather than Gi/Go and thus stimulates rather than inhibits adenylyl cyclase (Tong and Kish, unpublished results; reference 20).
Samples were first pre-incubated with the assay mixture on ice for 10 minutes and the reaction initiated by addition of ATP. The assay was carried out at 30°C for 30 minutes (dopamine stimulation) or 20 minutes (Gpp[NH]p stimulation). The assay was terminated by the addition of 2% sodium dodecylsulfate, 40 mM ATP, and 1.4 mM cAMP, followed by boiling for 10 minutes. Adenylyl cyclase activity was determined by the Dowex/Alumina two-column chromatography procedure of Salomon et al. (21) as described (19). Assays were carried out in duplicate, and activity was expressed as pmol cAMP formed per minute per mg of protein. Protein concentration was determined by using the Bio-Rad Protein Assay Kit with bovine plasma albumin as the standard.
Basal adenylyl cyclase activity was defined as the activity in the absence of any additional factors. Basal adenylyl cyclase with GTP was the activity in the presence of 10 μM GTP and was used for calculating percentage dopamine stimulation. The presence of GTP was required for the stimulation by dopamine and the concentration used maximally stimulated basal adenylyl cyclase activity (19). Gpp(NH)p stimulation was expressed as percentage increase above the basal adenylyl cyclase activity. The titration curves were fitted to the hyperbolic equation to calculate maximal stimulation and EC50 (the concentration producing 50% of the maximal stimulation), using Origin 5.0 (OriginLab Corporation, Northampton, Mass.).
Data Analysis
Statistical analyses were carried out with two-tailed Student’s t test for independent samples and one-way or two-way analyses of covariance (ANCOVA) with age and postmortem interval as the covariates followed by post hoc Tukey honestly significant difference tests. Pearson product-moment correlation or Spearman rank-order correlation analyses were used to examine the relationships indicated in the text.
Results
No significant correlations (Pearson) were found in either group between subject age and levels of any of the outcome measures. There were weak, negative correlations between postmortem interval and basal adenylyl cyclase activity in the absence or presence of GTP (comparison subjects: r=–0.35 to 0.01, df=19, p>0.11; methamphetamine users: r=–0.45 to –0.15, df=14, p>0.08) with that in frontal and temporal cortices of the comparison subjects being statistically significant (r=–0.58 to –0.45, df=19, p value range=0.005 to 0.04). For the comparison subjects (df=19), no significant correlations were found between postmortem interval and maximal dopamine stimulation in any of the examined brain areas (nucleus accumbens: r=–0.42, p=0.06; caudate: r=–0.33, p=0.14; putamen: r=0.15, p=0.51; frontal cortex: r=–0.29, p=0.20; temporal cortex: r=–0.23, p=0.31). No significant correlations were seen in the methamphetamine users (df=14) either between postmortem interval and maximal dopamine stimulation in any of the examined brain areas (nucleus accumbens: r=–0.15, p=0.57; caudate: r=–0.20, p=0.47; putamen: r=–0.03, p=0.92; frontal cortex: r=–0.26, p=0.33; temporal cortex: r=–0.20, p=0.46). A negative correlation was observed between postmortem interval and maximal Gpp(NH)p stimulation in all of the brain areas (comparison subjects: r=–0.59 to –0.36, df=19, p value range=0.004 to 0.11; methamphetamine users: r=–0.51 to –0.33, df=14, p value range=0.04 to 0.21), with significant correlations seen in the caudate (r=–0.59, df=19, p<0.005) and frontal cortex (r=–0.54, df=19, p<0.02) of the comparison subjects and in the nucleus accumbens of the methamphetamine users (r=–0.51, df=14, p<0.05).
As shown in Table 2, basal activity of adenylyl cyclase (in the presence of 10 μM GTP) was normal in all examined brain regions of the methamphetamine users. Dopamine titration (0.4–400 μM) in frontal cortex and striatum showed the expected dose dependence and was highly significant (F>200, df=7, 245, p<0.001) (Figure 1). No significant differences were observed in EC50 between the comparison subjects and the methamphetamine users (Table 2). However, the magnitude of maximal dopamine stimulation (calculated from the titration curves) in nucleus accumbens, caudate, and putamen of the methamphetamine users was significantly lower than those of the comparison subjects by 25%–30%, with nonsignificantly lower stimulation seen in the frontal cortex (17% decrease) and temporal cortex (20% decrease, calculated from a single saturating concentration of dopamine at 100 μM). As shown in Figure 2, although there was extensive overlap between the individual comparison and methamphetamine values for maximal dopamine stimulation, most of the methamphetamine user values in the striatum fell lower than the mean level for the comparison subjects.
Gpp(NH)p titration (10–8–10–4 M) was carried out in the nucleus accumbens, caudate, putamen, and frontal cortex. As described in Table 2, no significant difference was found between the methamphetamine users and comparison subjects in basal adenylyl cyclase activity, EC50, and the magnitude of Gpp(NH)p stimulation in any of the brain regions.
No significant correlations (Spearman rank) were found between the extent of maximal regional dopamine stimulation reported here and the regional protein concentrations of the dopamine D1 receptor in the 12 examined methamphetamine users in which both measurements were conducted (17), or between maximal dopamine stimulation and brain (occipital cortex) levels of methamphetamine plus its metabolite amphetamine in the entire group of 16 methamphetamine users (18) (data not shown). In addition, there was no significant correlation (Pearson) between dopamine stimulation and duration of drug use in those cases (N=11) for which accurate duration information was available (data not shown).
Discussion
The major finding of our study is that striatal dopamine D1-stimulated adenylyl cyclase activity is decreased in human chronic methamphetamine users.
We attempted to address, as much as possible, potential confounding issues associated with postmortem investigations of drug users. Thus, we obtained forensic evidence in blood, autopsied brain, and, for most of the users, hair, proving that the subjects of our study used methamphetamine both recently (drug positive in blood and brain) and chronically (sequential hair segments). Although the results of drug analyses and retrospective structured interviews suggested that the drug users used only methamphetamine, it is quite possible that the subjects might previously have used other drugs of abuse or might even have had a neurochemical defect before drug taking that could have affected the biochemical outcome measures. Issues surrounding differences in age, postmortem time, and agonal status (sudden versus slow death) were addressed by matching the comparison subjects and methamphetamine users with respect to these variables. We have also previously reported that the extent of maximal dopamine stimulation of adenylyl cyclase activity in biopsied versus autopsied human brain is similar (19). The absence of significant correlation between postmortem interval and dopamine stimulation was consistent with the reports on rats (22–24).
Our finding of decreased striatal dopamine stimulation of adenylyl cyclase in brain tissue of human methamphetamine users is consistent with investigations reporting below-normal dopamine D1 stimulation of striatal adenylyl cyclase following either acute administration (25–27) or repeated exposure plus drug challenge (28, 29) of amphetamine to rodents. The animal data suggest that impaired dopaminergic stimulation of adenylyl cyclase in humans was not a preexisting abnormality but rather a consequence of exposure to methamphetamine. However, repeated amphetamine administration to rodents can also induce enhanced dopamine D1 receptor-mediated inhibition of nucleus accumbens neurons (30, 31). Although the relevance of these experimental animal studies employing different treatment paradigms and outcome measures to human drug users is unknown (see reference 32 for review), this suggests that some dopamine D1 receptor-mediated functional changes might be differentially affected by methamphetamine.
The cause of the decreased dopamine stimulation of adenylyl cyclase in our human investigation is not known but in principle could be explained by decreased levels of or coupling between the dopamine receptor, G protein, and adenylyl cyclase. Our previous observations that striatal levels of dopamine D1 receptor protein are either normal (caudate, putamen) or elevated (nucleus accumbens) in methamphetamine users (17), together with our findings that levels of the stimulatory striatal G protein Golf(33) and basal adenylyl cyclase activity (present investigation) are normal, suggest that decreased dopaminergic stimulation of adenylyl cyclase is unlikely to be explained by low concentration (e.g., due to drug toxicity) of these components of the dopamine D1 receptor system. Similarly, the demonstration in methamphetamine-exposed rodents (25–29) and humans of normal adenylyl cyclase activation by activators (GTP or Gpp[NH]p), which directly stimulate adenylyl cyclase via Gs/Golf, suggests that G protein and adenylyl cyclase coupling is preserved and indicates specificity of the dopamine-related disturbance. However, the possibility cannot be excluded that G protein and adenylyl cyclase coupling associated with dopamine receptor activity might have represented only a minor part of total G protein and adenylyl cyclase coupling assessed under the conditions of our assay.
We suggest that desensitization of dopamine-stimulated adenylyl cyclase in the methamphetamine users could be explained by impaired coupling between the dopamine D1 receptor and the stimulatory G protein (27). Since all of the methamphetamine users used the drug recently as well as chronically (for at least 1 year), receptor desensitization might have occurred consequent to either acute or chronic drug exposure.
Although the relative biological importance of the different dopamine D1-linked effectors (e.g., adenylyl cyclase, phospholipase C [34]) is not known, it is reasonable to expect that much, if not most, of dopamine D1 receptor activity is mediated by G protein activation of adenylyl cyclase (12). Given that methamphetamine causes release of dopamine from striatal nerve endings, decreased dopamine-stimulated adenylyl cyclase activity in methamphetamine users can reasonably be considered as desensitization (i.e., biochemical tolerance) due to excessive dopaminergic stimulation of the dopamine D1 receptor. However, as the literature is limited on the nature of the involvement between dopamine D1 receptor function and drug-taking behavior in the human, the biological significance of impaired dopamine D1 receptor function in methamphetamine users is uncertain. In this regard, in view of the preliminary evidence that the dopamine D1 receptor might mediate part of the euphoric effects of dopaminergic psychostimulants in humans, decreased dopamine D1 receptor function in a limbic brain area (nucleus accumbens) suggests that some D1 receptor-related tolerance to the euphoric effects of methamphetamine might occur in human users following repeated drug exposure. It is also possible that subnormal dopamine D1 receptor-adenylyl cyclase activity in this brain area, in addition to the even more severe reduction in tissue stores of striatal dopamine (9, 10), might explain part of the dysphoria associated with withdrawal from methamphetamine. Finally, the preliminary report describing decreased craving for the psychostimulant cocaine in human users following administration of a selective D1 agonist (14) also suggests the much more speculative possibility that decreased dopamine D1-stimulated adenylyl cyclase activity in limbic brain could explain, in part, compulsive drug craving, which can occur in some chronic methamphetamine users (1).
Previously, we reported that concentrations of the dopamine D1 receptor were selectively increased in the postmortem nucleus accumbens subdivision of the striatum of 12 of the 16 methamphetamine users examined in the present study (17). We now find, however, that despite the increased receptor levels, a functional, and probably more biologically relevant, index of dopamine D1 receptor activity is below normal in the nucleus accumbens as well as in two other subdivisions in which dopamine D1 receptor number was normal. This discrepancy between receptor number and function suggests that caution should be employed in predicting functional changes in receptor activity from differences in receptor and G protein concentration. In this regard, the previous reports of changes in several components of the dopamine D2 receptor system in brain tissue of methamphetamine users, e.g., a trend for decrease in D2 receptor protein levels (17, 35) and decreased inhibitory G protein (33), need to be extended to include assessment of whether these changes actually affect D2 receptor-mediated activity. Unfortunately, however, because of high intersubject variability in our postmortem brain study, we were unable to develop a valid procedure that could assay dopamine D2 receptor-inhibited adenylyl cyclase activity in autopsied brain homogenates (Tong and Kish, unpublished observations).
We suspect that changes in activity of different dopamine (e.g., D1, D2) and nondopamine receptor systems underlie some of the behavioral effects of psychostimulant drugs. Our data provide the first functional data in human brain suggesting that the dopamine D1 receptor might be one of the systems involved in mediating as yet undetermined aspects of methamphetamine-induced behavior. Clinical studies of pharmacological agents modifying dopamine D1 receptor function in methamphetamine users will establish the biological significance of our neurochemical findings.
![]() |
![]() |
Received Feb. 11, 2002; revisions received Aug. 6 and Oct. 29, 2002; accepted Nov. 5, 2002. From the Human Neurochemical Pathology Laboratory and the Movement Disorder Research Laboratory, Center for Addiction and Mental Health; the Highland Psychiatric Research Foundation, Inverness, Scotland; the Office of the Santa Clara County Medical Examiner-Coroner, San Jose, Calif.; the Arkansas State Crime Laboratory, Little Rock; the Division of Forensic Toxicology, Armed Forces Institute of Pathology, Washington, D.C.; the Division of Neuropathology, London Health Science Center, University of Western Ontario, London, Ontario; the Spokane County Medical Examiner’s Office, Spokane, Wash.; and the Clark County Office of the Medical Examiner, Vancouver, Wash. Address reprint requests to Dr. Kish, Human Neurochemical Pathology Laboratory, Center for Addiction and Mental Health, 250 College St., Toronto, Ontario M5T 1R8, Canada; [email protected] (e-mail). Supported by National Institute on Drug Abuse grant DA-07182 to Dr. Kish. The opinions and assertions contained herein are the private views of the authors and are not to be construed as official or as reflecting views of the United States Department of Army or Department of Defense.
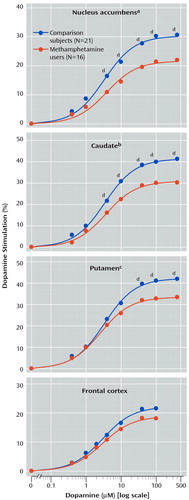
Figure 1. Dopamine Stimulation of Adenylyl Cyclase Activity in Postmortem Brain Tissue of Methamphetamine Users and Comparison Subjects
aSignificant difference between groups (F=12.5, df=1, 33, p<0.002).
bSignificant difference between groups (F=18.0, df=1, 33, p=0.0002).
cSignificant difference between groups (F=6.6, df=1, 33, p<0.02).
dSignificant post hoc difference between groups (p<0.004, Tukey honestly significant difference test).
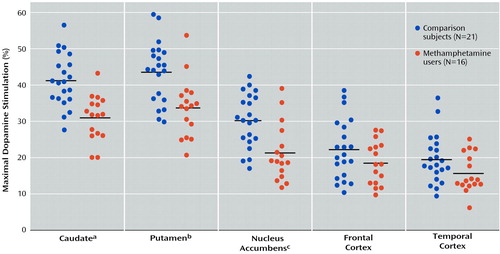
Figure 2. Maximal Extent of Dopamine Stimulation of Adenylyl Cyclase Activity in Postmortem Brain Tissue of Methamphetamine Users and Comparison Subjects
Significant difference between groups (F=22.3, df=1, 33, p<0.001).
aSignificant difference between groups (F=13.4, df=1, 33, p=0.001).
bSignificant difference between groups (F=12.3, df=1, 33, p=0.001).
1. Konuma K: Use and abuse of amphetamines in Japan, in Amphetamine and Its Analogs: Psychopharmacology, Toxicology, and Abuse. Edited by Cho AK, Segal DS. San Diego, Academic Press, 1994, pp 415-435Google Scholar
2. Cho AK, Melega WP: Patterns of methamphetamine abuse and their consequences. J Addict Dis 2002; 21:21-34Crossref, Medline, Google Scholar
3. Perez-Reyes M, White WR, McDonald SA, Hicks RE, Jeffcoat AR, Hill JM, Cook CE: Clinical effects of daily methamphetamine administration. Clin Neuropharmacol 1991; 14:352-358Crossref, Medline, Google Scholar
4. Di Chiara G, Imperato A: Drugs abused by humans preferentially increase synaptic dopamine concentrations in the mesolimbic system of freely moving rats. Proc Natl Acad Sci USA 1988; 85:5274-5278Crossref, Medline, Google Scholar
5. Laruelle M, Abi-Dargham A, van Dyck CH, Rosenblatt W, Zea-Ponce Y, Zoghbi SS, Baldwin RM, Charney DS, Hoffer PB, Kung HF, Innis RB: SPECT imaging of striatal dopamine release after amphetamine challenge. J Nucl Med 1995; 36:1182-1190Medline, Google Scholar
6. Volkow ND, Wang GJ, Fowler JS, Logan J, Gatley SJ, Wong C, Hitzemann R, Pappas NR: Reinforcing effects of psychostimulants in humans are associated with increases in brain dopamine and occupancy of D2 receptors. J Pharmacol Exp Ther 1999; 291:409-415Medline, Google Scholar
7. Drevets WC, Gautier C, Price JC, Kupfer DJ, Kinahan PE, Grace AA, Price JL, Mathis CA: Amphetamine-induced dopamine release in human ventral striatum correlates with euphoria. Biol Psychiatry 2001; 49:81-96Crossref, Medline, Google Scholar
8. Berke JD, Hyman SE: Addiction, dopamine, and the molecular mechanisms of memory. Neuron 2000; 25:515-532Crossref, Medline, Google Scholar
9. Wilson JM, Kalasinsky KS, Levey AI, Bergeron C, Reiber G, Anthony RM, Schmunk GA, Shannak K, Haycock JW, Kish SJ: Striatal dopamine nerve terminal markers in human, chronic methamphetamine users. Nat Med 1996; 2:699-703Crossref, Medline, Google Scholar
10. Moszczynska A, Guttman M, Kish SJ: Preferential loss of dopamine in caudate versus putamen of human methamphetamine users: possible relationship to specific features of drug withdrawal. Parkinsonism Relat Disord 2001; 7(suppl):S14Google Scholar
11. Zhuang X, Belluscio L, Hen R: Golfalpha mediates dopamine D1 receptor signaling. J Neurosci 2000; 20(RC91):1-5Google Scholar
12. Missale A, Nash SR, Robinson SW, Jaber M, Caron MG: Dopamine receptors: from structure to function. Physiol Rev 1998; 78:189-225Crossref, Medline, Google Scholar
13. Romach MK, Glue P, Kampman K, Kaplan HL, Somer GR, Poole S, Clarke L, Coffin V, Cornish J, O’Brien CP, Sellers EM: Attenuation of the euphoric effects of cocaine by the dopamine D1/D5 antagonist ecopipam (SCH 39166). Arch Gen Psychiatry 1999; 56:1101-1106Crossref, Medline, Google Scholar
14. Haney M, Collins ED, Ward AS, Foltin RW, Fischman MW: Effect of a selective dopamine D1 agonist (ABT-431) on smoked cocaine self-administration in humans. Psychopharmacology (Berl) 1999; 143:102-110Crossref, Medline, Google Scholar
15. Weed MR, Woolverton WL: The reinforcing effects of dopamine D1 receptor agonists in rhesus monkeys. J Pharmacol Exp Ther 1995; 275:1367-1374Medline, Google Scholar
16. Grech DM, Spealman RD, Bergman J: Self-administration of D1 receptor agonists by squirrel monkeys. Psychopharmacology (Berl) 1996; 125:97-104Crossref, Medline, Google Scholar
17. Worsley JN, Moszczynska A, Falardeau P, Kalasinsky KS, Schmunk G, Guttman M, Furukawa Y, Ang L, Adams V, Reiber G, Anthony RA, Wickham D, Kish SJ: Dopamine D1 receptor protein is elevated in nucleus accumbens of human, chronic methamphetamine users. Mol Psychiatry 2000; 5:664-672Crossref, Medline, Google Scholar
18. Kalasinsky KS, Bosy TZ, Schmunk GA, Reiber G, Anthony RM, Furukawa Y, Guttman M, Kish SJ: Regional distribution of methamphetamine in autopsied brain of chronic human methamphetamine users. Forensic Sci Int 2001; 116:163-169Crossref, Medline, Google Scholar
19. Tong J, Ross BM, Sherwin AL, Kish SJ: Dopamine D1-stimulated adenylyl cyclase activity in cerebral cortex of autopsied human brain. Neurochem Int 2001; 39:117-125Crossref, Medline, Google Scholar
20. Cowburn RF, O’Neill C, Ravid R, Winblad B, Fowler CJ: Preservation of Gi-protein inhibited adenylyl cyclase activity in the brains of patients with Alzheimer’s disease. Neurosci Lett 1992; 141:16-20Crossref, Medline, Google Scholar
21. Salomon Y, Londos C, Rodbell M: A highly sensitive adenylate cyclase assay. Anal Biochem 1974; 58:541-548Crossref, Medline, Google Scholar
22. Clement-Cormier YC, Kebabian JW, Petzold GL, Greengard P: Dopamine-sensitive adenylate cyclase in mammalian brain: a possible site of action of antipsychotic drugs. Proc Natl Acad Sci USA 1974; 71:1113-1117Crossref, Medline, Google Scholar
23. Carenzi A, Gillin JC, Guidotti A, Schwartz MA, Trabucchi M, Wyatt RJ: Dopamine-sensitive adenylyl cyclase in human caudate nucleus: a study in control subjects and schizophrenic patients. Arch Gen Psychiatry 1975; 32:1056-1059Crossref, Medline, Google Scholar
24. Nicol SE, Senogles SE, Caruso TP, Hudziak JJ, McSwigan JD, Frey WH: Postmortem stability of dopamine-sensitive adenylate cyclase, guanylate cyclase, ATPase, and GTPase in rat striatum. J Neurochem 1981; 37:1535-1539Crossref, Medline, Google Scholar
25. Barnett JV, Kuczenski R: Desensitization of rat striatal dopamine-stimulated adenylate cyclase after acute amphetamine administration. J Pharmacol Exp Ther 1986; 237:820-825Medline, Google Scholar
26. Roberts-Lewis JM, Roseboom PH, Iwaniec LM, Gnegy ME: Differential down-regulation of D1-stimulated adenylate cyclase activity in rat forebrain after in vivo amphetamine treatments. J Neurosci 1986; 6:2245-2251Crossref, Medline, Google Scholar
27. Roseboom PH, Gnegy ME: Acute in vivo amphetamine produces a homologous desensitization of dopamine receptor-coupled adenylate cyclase activities and decreases agonist binding to the D1 site. Mol Pharmacol 1989; 35:139-147Medline, Google Scholar
28. Barnett JV, Segal DS, Kuczenski R: Repeated amphetamine pretreatment alters the responsiveness of striatal dopamine-stimulated adenylate cyclase to amphetamine-induced desensitization. J Pharmacol Exp Ther 1987; 242:40-47Medline, Google Scholar
29. Roseboom PH, Hewlett GH, Gnegy ME: Repeated amphetamine administration alters the interaction between D1-stimulated adenylyl cyclase activity and calmodulin in rat striatum. J Pharmacol Exp Ther 1990; 255:197-203Medline, Google Scholar
30. Higashi H, Inanaga K, Nishi S, Uchimura N: Enhancement of dopamine actions on rat nucleus accumbens neurones in vitro after methamphetamine pre-treatment. J Physiol 1989; 408:587-603Crossref, Medline, Google Scholar
31. Wolf ME, White FJ, Hu XT: MK-801 prevents alterations in the mesoaccumbens dopamine system associated with behavioral sensitization to amphetamine. J Neurosci 1994; 14:1735-1745Crossref, Medline, Google Scholar
32. Brauer LH, Goudie AJ, de Wit H: Dopamine ligands and the stimulus effects of amphetamine: animal models versus human laboratory data. Psychopharmacology (Berl) 1997; 130:2-13Crossref, Medline, Google Scholar
33. McLeman ER, Warsh JJ, Ang L, Li PP, Kalasinsky KS, Ross BM, Tong J, Schmunk G, Adams V, Kish SJ: The human nucleus accumbens is highly susceptible to G protein down-regulation by methamphetamine and heroin. J Neurochem 2000; 74:2120-2126Crossref, Medline, Google Scholar
34. Undie AS, Friedman E: Stimulation of a dopamine D1 receptor enhances inositol phosphates formation in rat brain. J Pharmacol Exp Ther 1990; 253:987-992Medline, Google Scholar
35. Volkow ND, Chang L, Wang G-J, Fowler JS, Ding Y-S, Sedler M, Logan J, Franceschi D, Gatley J, Hitzemann R, Gifford A, Wong C, Pappas N: Low level of brain dopamine D2 receptors in methamphetamine abusers: association with metabolism in the orbitofrontal cortex. Am J Psychiatry 2001; 158:2015-2021Link, Google Scholar