Effect of Impaired Recognition and Expression of Emotions on Frontocingulate Cortices: An fMRI Study of Men With Alexithymia
Abstract
OBJECTIVE: Although the brain areas involved in emotional response and in the recognition of others’ emotions have been reported, the neural bases of individual differences in affective style remain to be elucidated. Alexithymia, i.e., impairment of the ability to identify and communicate one’s emotional state, influences how emotions are regulated. Alexithymia has been hypothesized to involve anterior cingulate dysfunction. Therefore, the authors searched for differential cerebral regional activation in response to emotional stimuli in subjects with alexithymia. METHOD: Two groups of eight men each were selected from 437 healthy subjects on the basis of high or low scores on the 20-item Toronto Alexithymia Scale. Using functional magnetic resonance imaging (fMRI), the authors compared the two groups for their regional cerebral activation in response to the presentation of pictures with validated positive or negative arousal capabilities. RESULTS: Men with alexithymia demonstrated less cerebral activation in the left mediofrontal-paracingulate cortex in response to highly negative stimuli and more activation in the anterior cingulate, mediofrontal cortex, and middle frontal gyrus in response to highly positive stimuli than men without alexithymia. CONCLUSIONS: These findings provide direct evidence that alexithymia, a personality trait playing a role in affect regulation, is linked with differences in anterior cingulate and mediofrontal activity during emotional stimuli processing.
The brain areas involved in positive and negative emotion and in the recognition of emotional expressions of others have been revealed by neuroimaging studies in normal subjects and in subjects with dysfunctional states (e.g., neurological or mood disorders) (e.g., references 1–4). However, the neural bases of individual differences in affective style remain to be elucidated (5). To our knowledge, only one functional magnetic resonance imaging (fMRI) study (6) showed that personality is associated with brain reactivity to emotional stimuli. This issue constitutes a challenge because dispositional differences in affectivity influence how emotions are regulated and may account for the vulnerability to psychopathology.
The ability to identify and communicate one’s feelings is a personality trait that differs among people (7). About 10% of the normal population are characterized by poor expressiveness of emotional states, i.e., alexithymia (8). Alexithymia is a subclinical phenomenon marked by the following impairments: difficulty in identifying and describing feelings, difficulty in distinguishing feelings from the bodily sensations of emotional arousal, impaired symbolization, and a tendency to focus on external events rather than inner experiences (9). Moreover, subjects with alexithymia tend to avoid conflicts and to rely on action to express emotion and anxiety; they are socially conforming and humorless and experience meaninglessness (10). This condition is considered a disorder of affect regulation (11). Alexithymia is a risk factor for several somatic disorders (e.g., chronic pain, breast cancer) and psychiatric disorders (e.g., substance-related and eating disorders) (12), and it has also been associated with a higher risk of mortality in middle-aged men (13). Direct evidence that this personality trait is associated with impaired processing of emotional stimuli is limited to electrophysiological studies on autonomic arousal and experimental psychology studies showing impaired facial expression recognition or processing of emotional words or scenes. Alexithymia has been associated in some studies (14–19) with a high and stable level of autonomic reactivity at baseline but not in other studies (20, 21). Moreover, alexithymia has been associated with hyperarousal to emotional stimuli or situational stressors (15, 17, 22) and, contradictorily, with less reactivity (19, 21, 23, 24) or similar reactivity (14, 16, 18). However, the question remains as to whether alexithymia is associated with differences in brain activation during emotional responding.
Lane et al. (25) attempted to identify brain regions associated with conscious experience of emotion by a correlation analysis between blood flow changes induced by emotional stimuli and scores on the Level of Emotional Awareness Scale (26). The area of maximum correlation was located in the cingulate cortex. On the basis of findings that emotional awareness is correlated with blood flow in the anterior cingular cortex (25) and that the anterior cingular cortex is one of the structures involved in emotional experience (3), Lane et al. (27) speculated that alexithymia would be associated with a deficit in the participation of the anterior cingular cortex during emotional arousal. They conceptualized alexithymia as the emotional equivalent of blindsight and coined the term “blindfeel” (27).
To investigate this model, we attempted to demonstrate that differences in the ability to identify and describe one’s feelings are associated with differences in neural substrates involved in processing emotional stimuli. We tested this hypothesis in a comparative study of the cerebral regions activated during passive viewing of emotional stimuli. We postulated that the differences would involve structures implicated in higher-level processing of the emotional significance of complex stimuli, i.e., the anterior cingulate and mediofrontal gyri. Indeed, the medial prefrontal and paracingulate cortices are the sites of convergence for limbic inputs. These regions may participate in integrating cognition and emotion, particularly in the processing of affect-related meanings (4, 28) and in the representation of the mental states of others (29).
Functional imaging also suggests that the medial prefrontal and anterior cingular cortices are involved in representing subjective emotional inner states (30, 31). These regions have been postulated to participate in the conscious experience of emotion (25, 32), the inhibition of potentially excessive emotion, and the regulation of emotional expression (3).
However, the question of whether alexithymia is associated with hyporesponsiveness or hyperresponsiveness to emotional stimuli is still controversial. Because the anterior cingular cortex is considered a superordinate structure that can facilitate some processes but also suppress others (33, 34), there is no unequivocal support for the prediction of directional effects in the group comparison.
Method
Participants
A screening procedure was used to select study participants on the basis of their ability to identify and describe their feelings. Right-handed, nonanxious, nondepressed (based on Hospital Anxiety and Depression Scale scores [35]) men were recruited from a group 437 students according to their alexithymia scores on the 20-item Toronto Alexithymia Scale (36, 37). The Toronto Alexithymia Scale, an extensively validated self-report alexithymia questionnaire, is the most commonly used scale to assess alexithymia. The scale is clustered into factors theoretically congruent with the alexithymia construct. Two factors correspond to the affective disturbance described by Nemiah and Sifneos (38) (i.e., factor 1 is difficulty in identifying and distinguishing between feelings and bodily sensations and factor 2 is difficulty in describing feelings). Scores on these factors were summed. The subjects with and without alexithymia were selected, respectively, from the first and the last quartile of the initial distribution of scores on Toronto Alexithymia Scale factor 1 plus factor 2. In Toronto Alexithymia Scale total scores, the subjects selected for the two groups fulfilled the criteria for either presence or absence of alexithymia. (Cutoff scores for the French 20-item Toronto Alexithymia Scale [39] are 56 and above for presence and 44 or below for absence of alexithymia.)
The 16 subjects who were selected filled in the Toronto Alexithymia Scale as well as the Hospital Anxiety and Depression Scale again on the study day to make sure that they were not depressed and not anxious and that their Toronto Alexithymia Scale scores were stable.
Eight men with and eight men without alexithymia (mean age=21.5 years) were recruited by following this procedure (Table 1). None of the men had a history of medical, neurological, or psychiatric disorder. After complete description of the study to the subjects, written informed consent was obtained. The local ethics committee approved the study. The participants received course credit for their participation.
Experimental Design
Figure 1 presents an outline of the experimental design. The stimuli were five sets of 12 pictures selected from the International Affective Picture System (40) according to their pleasantness (positive, negative, or neutral valence) and emotional intensity (high or low arousal) on the basis of International Affective Picture System norms (Table 2). The five sets showed pictures that had positive valence and high-arousal intensity, positive valance and low-arousal intensity, negative valence and high-arousal intensity, negative valence and low-arousal intensity, and neutral valence and neutral intensity.
Control stimuli were created by scrambling the initial pictures to suppress their emotional tenor. Cerebral activation in response to pictures that were positive, negative, or neutral was contrasted with that for their corresponding control stimuli.
There were four runs, each consisting of alternated presentations of blocks of three pictures with constant emotional valence and constant arousal and their three corresponding control pictures. Each picture and its control was presented for 6 seconds (one block=18 seconds), with no interval between the blocks. The order of the blocks was counterbalanced across the runs and across the subjects. The runs started by presenting four control pictures that were excluded from the analyses.
A mirror allowed the men to view visual stimuli that were rear-projected on a 100-by-80-cm screen. Stimuli were 55 cm wide by 70 cm high when projected on the screen. Pictures were presented under computer control and synchronized with the start of the scanner.
Subjects were informed that some pictures might be shocking. To assess spontaneous reaction, no explicit evaluation of the emotional valence of the stimuli was required during the scans. Participants were instructed to maintain their attention as long as the stimuli were displayed.
Subjects’ ratings of the pleasantness of the positive pictures and the unpleasantness of the negative pictures were recorded immediately after the scanning session. Pictures of each type were rated as a group from memory. Two nongraduated visual analogue scales were used (from neutral to extremely pleasant and from neutral to extremely unpleasant).
Image Acquisition and Data Analysis
MRI data were acquired on a Medspec Avance system (Bruker Medical, Ettlingen, Germany) using a 3-T whole-body magnet and an actively shielded 33-cm gradient insert capable of producing a 25-mT/m gradient with a 150-second ramp time. A quadrature birdcage resonator of 25-cm inner diameter was used for excitation and detection. Functional blood-oxygen-level-dependent-contrast MRI was performed with an echo-planar image sequence, a 64×80 matrix, and a 24×30-cm field of view. The rectangular field of view was used to better separate the N/2-ghost artifact from the original image. Twenty contiguous axial slices of 6-mm thickness were acquired with a repetition time of 2 seconds and a tip angle of 90°. Nine scans per block were acquired. No signal averaging was performed. Distortions of echo-planar images caused by field inhomogeneity were corrected (41). The anatomical reference data were acquired by using an inversion-recovery-prepared three-dimensional sequence of fast low-angle shots (TI=600 msec, TR=2 seconds). A matrix size of 256×256×128, field of view of 256 mm, and a single accumulation were used. We used statistical parametric mapping (SPM 99, Wellcome Department of Cognitive Neurology, Institute of Neurology, University College, London) to realign images of all subjects to correct for artifacts caused by small head movements and adjusted these into Talairach and Tournoux stereotaxic space (42). Images were then smoothed with a three-dimensional isotropic gaussian kernel (7-mm full width at half maximum) to improve signal-to-noise ratio.
For statistical analysis, time series of the images were correlated with a box-car function convoluted with an estimation of the hemodynamic response, which approximates the activation patterns, and a linear model for autocorrelated observations was applied voxel-wise (43). Low frequency was filtered out by using a high-pass filter with cutoff frequency of 1/216 Hz.
Regional specific effects were assessed in terms of t values. For each experimental condition, differences were assessed between alexithymic and nonalexithymic subjects in cerebral regional activation in response to pictures compared with scrambled pictures (their controls). The analysis was based on the interaction of positive high-arousal and low-arousal pictures and their controls; negative high-arousal and low-arousal pictures and their controls, and neutral pictures and their controls. Neutral stimuli were used as a reference condition; no between-group differences were expected in this condition.
Regional activation was assessed for each subject. T maps comparing these activations in alexithymic and nonalexithymic groups were computed by using SPM 99. Following random-effect-analysis procedure, we computed the variance across subjects, allowing generalization of the results (44). To reduce the risk of false negatives, we used a hypothesis-driven approach centered on the anterior cingular cortex and medial prefrontal cortices; the height and extent of statistical thresholds were set to z>3.09 and seven voxels, respectively, and p<0.001 and p<0.05, respectively, uncorrected. This approach is known to be more stringent than relying on a corrected p value and using a fixed-effect analysis. These thresholds have been chosen in many previous imaging studies (e.g., reference 45).
Results
Subjective Ratings
T tests showed no group effect on the mean subjective ratings of the pleasantness or unpleasantness of the positive or negative pictures. Mean scores of the alexithymic and nonalexithymic groups were, respectively, 6.31 (SD=1.61) and 6.70 (SD=2.25) for positive pictures (F=1.34, df=1, 14, n.s.) and 6.28 (SD=1.59) and 6.12 (SD=1.87) for negative pictures (F<1, df=1, 14, n.s.).
fMRI Results
At a threshold of p<0.001 (uncorrected) for statistical significance, no difference in response to neutral stimuli or to emotional stimuli with low arousal was detected between alexithymic and nonalexithymic groups. However, the two groups showed different patterns of activation for the emotional stimuli with high arousal.
Negative high-arousal stimuli (negative high-arousal pictures compared with their controls) induced less activation in men with alexithymia than in those without alexithymia in the left mediofrontal-paracingulate gyrus. Conversely, positive high-arousal stimuli (positive high-arousal pictures compared with their controls) were associated with greater activation bilaterally in the anterior cingulate, mediofrontal, and middle frontal gyri in men with alexithymia than in those without alexithymia. These were the only regions showing between-group differences at this level of significance (coordinates and z scores are given in Table 3). The results of statistical parametric mapping and the associated adjusted responses for the between-group comparisons in the positive and negative high-arousal experimental conditions at the maximal voxel of activation are presented in Figure 2.
Between-group differences were observed in the positive and negative low-arousal conditions, but not in the neutral condition, at a lower level of significance (height and extent thresholds, respectively, set to p<0.005 and p<0.05, uncorrected). Negative low-arousal pictures induced greater signal increases in alexithymic than in nonalexithymic groups bilaterally in the middle frontal gyrus (Brodmann’s area 9), the left superior and inferior parietal lobule (Brodmann’s area 7/40 and Brodmann’s area 40, respectively), and the left middle temporal gyrus (Brodmann’s area 21). Positive low-arousal pictures induced greater signal increases in alexithymic than in nonalexithymic groups in the left middle temporal gyrus (Brodmann’s area 21), the right inferior parietal lobule (Brodmann’s area 39), and bilaterally in the superior parietal lobule (Brodmann’s area 7).
Discussion
The main results of this study demonstrate differences between men with and without alexithymia (defined as an inability to identify and communicate one’s feelings) in their neural response to emotional stimuli. The anterior cingulate and mediofrontal cortices were differently activated by intense emotional stimuli (negative or positive pictures) in men with and without the disorder. Conversely, men with and without alexithymia showed similar patterns of brain activity in the reference experimental condition (neutral pictures).
Previous brain imaging studies that attempted to test whether individual differences in affectivity are associated with differential brain reactivity to emotional stimuli relied on correlation analyses between personality scores and degree of activation in localized brain regions (6, 25). However, participants in these studies had personality scores within the average range of a normative sample. In contrast, the present experiment involved a stringent screening procedure. The eight men with and eight without alexithymia had scores at the extreme ends of scores among a large group of subjects (N=437). This procedure enabled us to compare the two groups.
Our observation of a differential response predominantly in the anterior cingular and mediofrontal cortices between men with and without alexithymia indicates that alexithymia may be linked to structures involved in the appraisal of the emotional content of the stimuli rather than in lower levels of processing during the passive viewing of these stimuli. Consistent with the suggestion that alexithymia is associated with a deficit in the cognitive evaluation of emotion, no between-group difference was observed in limbic structures (i.e., the amygdala, the hippocampal formation, and the hypothalamus), which play a central role in emotional response to simple perceptual and associative aspects of the stimuli but may be less relevant for their interpretation (46).
The fact that no difference was found between groups while they were processing neutral pictures further supports the hypothesis that alexithymia is a deficit involving emotional processing. Indeed, using control stimuli as a contrast condition and using neutral pictures as a reference condition rather than as a contrast condition allowed us to verify that between-group differences were not due to experimental environment (e.g., group differences in anxiety induced by the MRI confinement) but were specific to the processing of emotional stimuli. Furthermore, the fact that no explicit recognition or categorization of emotional valence was required during the scanning session in our experimental design suggests that the differences were not contingent on introspective attentional effort but were related to spontaneous emotional experience.
To our knowledge, these results provide the first set of functional brain imaging data in favor of the “blindfeel” model, which postulates impaired anterior cingular cortex functioning in alexithymia (27). However, our results are only partially in line with this model, which does not predict an effect of valence. Indeed, we observed a differential activation for stimuli of positive and negative valence. Mediofrontal and anterior cingular cortex structures were less activated by intense negative stimuli in alexithymic than in nonalexithymic men, but these areas were more activated by intense positive stimuli in alexithymic than in nonalexithymic men. Besides, between-group differences for negative and positive pictures occurred in adjacent regions: in rostral cingular/mediofrontal regions with negative pictures and in the dorsal anterior cingular cortex with positive pictures.
As mentioned at the beginning of this article, both mediofrontal and anterior cingulate cortices have been postulated to participate in the experience of emotion and affect regulation. It is also noteworthy that the anterior cingular cortex has been functionally divided into a dorsal division and a rostral-ventral division (33, 47). These two areas of the anterior cingular cortex might participate in different aspects of emotional experience (48). The dorsal anterior cingular cortex may be involved in the direct experience of an emotion (“phenomenal awareness”), whereas the rostral-ventral part may be involved in the capacity to reflect on the contents of phenomenal awareness and establish a representation of emotional state (“reflective awareness”) (48).
Although our findings should be considered preliminary, we speculate that the increase of activity observed in the dorsal anterior cingular cortex in subjects with alexithymia when they view positive pictures suggests that alexithymia is associated with enhanced “phenomenal awareness” of positive affect. Additionally, the decrease of activity in the rostral anterior cingular cortex/mediofrontal cortex when viewing negative pictures suggests that alexithymia is associated with a deficit in “reflective awareness” (i.e., representation of the current emotional state) of negative affect. This double emotional dysregulation could account for the fact that subjects with alexithymia have vague emotional feelings that cannot be differentiated.
With respect to the subjects’ self-ratings after the scan, which relied on nonverbal visual analogue scales, the pleasantness and unpleasantness of the positive and negative pictures were rated similarly by men with and without alexithymia. This finding is consistent with the literature showing, first, that subjects with alexithymia often have accurate judgments of affect-laden external stimuli and, second, that in the context of experimentally induced emotion, they have a similar self-reported emotional state when coarse rating methods are used (see reference 21). However, when more targeted self-report measures are used, such as having the subject describe how the stimuli made them feel (19, 21) (see also reference 27) or relying on graphic depictions to describe the induced emotional state (21), impoverished affective self-description is observed in subjects with alexithymia.
Conclusions
The present study offers evidence that alexithymia, a personality trait playing a role in affect regulation, is associated with circumscribed valence-dependent differences in activity in the anterior cingular and mediofrontal cortices during emotional stimuli processing. Previous research in the field of affective neuroscience and functional imaging studies on emotions in humans have either examined how clinically disturbed groups differ in processing emotional information or have used experimental manipulation of mood states in normal subjects. From our results, it appears that dispositional differences in affectivity may also constitute relevant variables to consider.
![]() |
![]() |
![]() |
Presented in part at the annual meeting of the Society of Biological Psychiatry, Chicago, May 11–13, 2000, and at the sixth annual meeting of the Organization for Human Brain Mapping, San Antonio, Tex., June 12–16, 2000. Received May 30, 2001; revision received Jan. 10, 2002; accepted Jan. 16, 2002. From the Equipe de Recherche ERM 0205, “Imagerie Cérébrale en Psychiatrie,” Institut National de la Santé et de la Recherche Médicale (INSERM), Service Hospitalier Frédéric Joliot–Commissariat à l’Energie Atomique, Orsay, France; the Department of Psychiatry, Institut Mutualiste Montsouris, Paris; and the Department of Psychiatry, Hôpital Européen Georges Pompidou, Paris. Address reprint requests to Dr. Martinot, INSERM U334, SHFJ/CEA, 4 Place du Général Leclerc, 91401 Orsay Cedex, France; [email protected] (e-mail). Supported by a grant from the French groupement d’Intérêt Scientifique “Cognitique.”
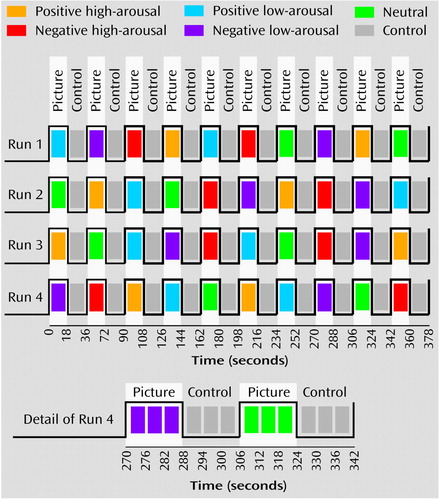
Figure 1. Order of Presentation of Pictures With Varying Valence and Arousal in fMRI Study of Emotional Recognition and Expression in Alexithymia
aFive sets of 12 pictures were selected from the International Affective Picture System (40) according to their pleasantness (positive, negative, or neutral valence) and emotional intensity (high or low arousal) on the basis of International Affective Picture System norms. Each run consisted of alternated presentations of blocks of three pictures with constant emotional valence and constant arousal and blocks of their corresponding control stimuli (scrambled versions of the initial pictures).
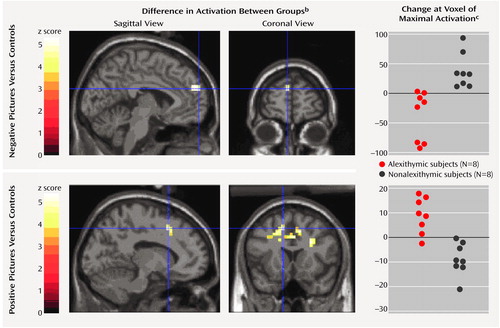
Figure 2. Brain Images Showing Differences Between Eight Men With and Eight Men Without Alexithymia in Activation in Response to Pictures With Negative or Positive Valencea and Associated Adjusted Responses at the Maximal Voxel of Activation
aFive sets of 12 pictures were selected from the International Affective Picture System (40) according to their pleasantness (positive, negative, or neutral valence) and emotional intensity (high or low arousal) on the basis of International Affective Picture System norms.
bThe bars on the left show the range of z scores for statistical parametric mapping of the comparisons showing differences in regional cerebral activation between men with and without alexithymia in response to contrasts of negative pictures versus their controls and positive pictures versus their controls. Views of the brain are shown for orthogonal slices at the voxel of maximal activation of the largest cluster in the mediofrontal gyrus. The sagittal views show the left mediofrontal gyrus.
cAdjusted changes in blood-oxygen-level-dependent activity at the voxel of maximal activation in the left mediofrontal-paracingulate gyrus (Talairach and Tournoux coordinates x=–4, y=60, z=24) for the negative pictures and in the left mediofrontal gyrus (Talairach and Tournoux coordinates x=–12, y=16, z=48) for the positive pictures. Values are mean-corrected across each group.
1. Andreasen NC: Linking mind and brain in the study of mental illnesses: a project for a scientific psychopathology. Science 1997; 275:1586-1593Crossref, Medline, Google Scholar
2. Blair RJR, Morris JS, Frith CD, Perrett DI, Dolan RJ: Dissociable neural responses to facial expressions of sadness and anger. Brain 1999; 122:100-111Crossref, Google Scholar
3. Reiman EM: The application of positron emission tomography to the study of normal and pathologic emotions. J Clin Psychiatry 1997; 58:4-12Medline, Google Scholar
4. Teasdale JD, Howard RJ, Cox SG, Ha Y, Brammer MJ, Williams SCR, Checkley SA: Functional MRI study of the cognitive generation of affect. Am J Psychiatry 1999; 156:209-215Abstract, Google Scholar
5. Davidson RJ, Irwin W: The functional neuroanatomy of emotion and affective style. Trends Cogn Sci 1999; 3:11-21Crossref, Medline, Google Scholar
6. Canli T, Zhao Z, Desmond JE, Kang E, Gross J, Gabrieli JDE: An fMRI study of personality influences on brain reactivity to emotional stimuli. Behav Neurosci 2001; 115:33-42Crossref, Medline, Google Scholar
7. Sifneos PE: Alexithymia: past and present (festschrift). Am J Psychiatry 1996; 153(July suppl):137-142Google Scholar
8. Linden W, Wen F, Paulhaus DL: Measuring alexithymia: reliability, validity, and prevalence, in Advances in Personality Assessment. Edited by Butcher J, Spielberger C. Hillsdale, NJ, Lawrence Erlbaum Associates, 1994, pp 125-143Google Scholar
9. Taylor GJ, Bagby RM, Parker JDA: The alexithymia construct: a potential paradigm for psychosomatic medicine. Psychosomatics 1991; 32:153-164Crossref, Medline, Google Scholar
10. Haviland MG, Reise SP: A California Q-set alexithymia prototype and its relationship to ego-control and ego-resiliency. J Psychosom Res 1996; 41:597-608Crossref, Medline, Google Scholar
11. Bagby RM, Taylor GJ: Affect dysregulation and alexithymia, in Disorders of Affect Regulation: Alexithymia in Medical and Psychiatric Illness. Edited by Taylor GJ, Bagby RM, Parker JDA. Cambridge, UK, Cambridge University Press, 1997, pp 26-45Google Scholar
12. Taylor GJ, Bagby RM, Parker JDA: Disorders of Affect Regulation: Alexithymia in Medical and Psychiatric Illness. Cambridge, UK, Cambridge University Press, 1997Google Scholar
13. Kauhanen J, Kaplan GA, Cohen RD, Julkunen J, Salonen T: Alexithymia and risk of death in middle-aged men. J Psychosom Res 1996; 41:541-549Crossref, Medline, Google Scholar
14. Friedlandler L, Lumley MA, Farchione T, Doyal G: Testing the alexithymia hypothesis: physiological and subjective responses during relaxation and stress. J Nerv Ment Dis 1997; 185:233-239Crossref, Medline, Google Scholar
15. Infrasca R: Alexithymia, neurovegetative arousal and alexithymia: an experimental study. Psychother Psychosom 1997; 66:276-280Crossref, Medline, Google Scholar
16. Martin JB, Pihl RO: Influence of alexithymic characteristics on physiological and subjective stress response in normal individuals. Psychother Psychosom 1986; 45:66-77Crossref, Medline, Google Scholar
17. Rabavilas AD: Electrodermal activity in low and high alexithymic neurotic patients. Psychother Psychosom 1987; 47:101-104Crossref, Medline, Google Scholar
18. Stone LA, Nielson KA: Intact physiological response to arousal with impaired emotional recognition in alexithymia. Psychother Psychosom 2001; 70:92-102Crossref, Medline, Google Scholar
19. Whemer F, Brejnak C, Lumley MA, Stettner L: Alexithymia and physiological reactivity to emotion-provoking visual scenes. J Nerv Ment Dis 1995; 183:351-357Crossref, Medline, Google Scholar
20. Newton TL, Contrada RJ: Alexithymia and repression: contrasting emotion-focused styles. Psychosom Med 1994; 56:457-462Crossref, Medline, Google Scholar
21. Roedema TM, Simons RF: Emotion-processing deficit in alexithymia. Psychophysiology 1999; 36:379-387Crossref, Medline, Google Scholar
22. Papciak AS, Feuerstein M, Spiegel JA: Stress reactivity in alexithymia: decoupling of physiological and cognitive responses. J Hum Stress 1985; 11:135-142Crossref, Medline, Google Scholar
23. Hyer L, Woods MG, Summers MN, Boudewyns P, Harrison WR: Alexithymia among Vietnam veterans with posttraumatic stress disorder. J Clin Psychiatry 1990; 51:243-247Medline, Google Scholar
24. Linden W, Lenz JW, Stossel C: Alexithymia, defensiveness and cardiovascular reactivity to stress. J Psychosom Res 1996; 41:575-583Crossref, Medline, Google Scholar
25. Lane RD, Reiman EM, Axelrod B, Lang-Sheng Y, Holmes A, Schwartz GE: Neural correlates of levels of emotional awareness: evidence of an interaction between emotion and attention in the anterior cingulate cortex. J Cogn Neurosci 1998; 10:525-535Crossref, Medline, Google Scholar
26. Lane RD, Schwartz GE: Levels of emotional awareness: a cognitive-developmental theory and its application to psychopathology. Am J Psychiatry 1987; 144:133-143; correction, 144:542Link, Google Scholar
27. Lane RD, Ahern GL, Schwartz GE, Kaszniak AW: Is alexithymia the emotional equivalent of blindsight? Biol Psychiatry 1997; 42:834-844Crossref, Medline, Google Scholar
28. Beauregard M, Chertkow H, Bub D, Murtha S, Dixon R, Evans R: The neural substrates for concrete, abstract, and emotional word lexica: a PET study. J Cogn Neurosci 1997; 9:441-461Crossref, Medline, Google Scholar
29. Happé F, Ehlers S, Fletcher PC, Johansson M, Gillberg C, Dolan RJ, Frackowiak RSJ, Frith CD: “Theory of mind” in the brain: evidence from a PET scan study of Asperger syndrome. Neuroreport 1996; 8:197-201Crossref, Medline, Google Scholar
30. Beauregard M, Leroux JM, Bergman S, Arzoumanian Y, Beaudoin G, Bourgouin P, Stip E: The functional neuroanatomy of major depression: an fMRI study using an emotional activation paradigm. Neuroreport 1998; 9:3253-3258Crossref, Medline, Google Scholar
31. Lane RD, Reiman EM, Bradley MM, Lang PJ, Ahern GL, Davidson RJ, Schwartz GE: Neuroanatomical correlates of pleasant and unpleasant emotion. Neuropsychologia 1997; 35:1437-1444Crossref, Medline, Google Scholar
32. Lane RD, Fink GR, Chau PM-L, Dolan RJ: Neural activation during selective attention to subjective emotional responses. Neuroreport 1997; 8:3969-3972Crossref, Medline, Google Scholar
33. Bush G, Luu P, Posner MI: Cognitive and emotional influences in anterior cingulate cortex. Trends Cogn Sci 2000; 4:215-222Crossref, Medline, Google Scholar
34. Paus T: Primate anterior cingulate cortex: where motor control, drive and cognition interface. Nat Rev Neurosci 2001; 2:417-423Crossref, Medline, Google Scholar
35. Zigmond AS, Snaith RP: The Hospital Anxiety and Depression Scale. Acta Psychiatr Scand 1983; 67:361-370Crossref, Medline, Google Scholar
36. Bagby RM, Parker JDA, Taylor GJ: The Twenty-Item Toronto Alexithymia Scale, I: item selection and cross-validation of the factor structure. J Psychosom Res 1994; 38:23-32Crossref, Medline, Google Scholar
37. Bagby RM, Taylor GJ, Parker JDA: The Twenty-Item Toronto Alexithymia Scale, II: convergent, discriminant, and concurrent validity. J Psychosom Res 1994; 38:33-40Crossref, Medline, Google Scholar
38. Nemiah JC, Sifneos PE: Psychosomatic illness: a problem in communication. Psychother Psychosom 1970; 18:154-160Crossref, Medline, Google Scholar
39. Loas G, Otmani O, Fremaux D, Lecercle C, Duflot M, Delahousse J: Etude de la validité externe, de la fidélité et détermination des notes seuils des échelles d’alexithymie de Toronto (TAS et TAS-20) chez un groupe de malades alcooliques. Encéphale 1996; 22:35-40Google Scholar
40. Lang PJ, Bradley MM, Cuthbert BN: International Affective Picture System (IAPS): Technical Manual and Affective Ratings. Gainesville, University of Florida, Center for Research in Psychophysiology, 1997Google Scholar
41. Jezzard P, Barnett AS, Pierpaoli C: Characterization of and correction for eddy current artifacts in echo planar diffusion imaging. Magn Reson Med 1998; 39:801-812Crossref, Medline, Google Scholar
42. Talairach J, Tournoux P: Co-Planar Stereotaxic Atlas of the Human Brain: Three-Dimensional Proportional System. New York, Thieme Medical, 1988Google Scholar
43. Worsley KJ, Friston KJ: Analysis of fMRI time-series revisited—again. Neuroimage 1995; 2:173-181Crossref, Medline, Google Scholar
44. Friston KJ, Holmes AP, Worsley KJ: How many subjects constitute a study? Neuroimage 1999; 10:1-5Crossref, Medline, Google Scholar
45. Morris JS, Frith CD, Perrett DI, Rowland D, Young AW, Calder AJ, Dolan RJ: A differential neural response in the human amygdala to fearful and happy facial expressions. Nature 1996; 383:812-815Crossref, Medline, Google Scholar
46. Reiman EM, Lane RD, Ahern GL, Schwartz GE, Davidson RJ, Friston KJ, Yun L-S, Chen K: Neuroanatomical correlates of externally and internally generated human emotion. Am J Psychiatry 1997; 154:918-925Link, Google Scholar
47. Devinsky O, Morrell MJ, Vogt BA: Contributions of anterior cingulate cortex to behavior. Brain 1995; 118:279-306Crossref, Medline, Google Scholar
48. Lane RD: Neural correlates of conscious emotional experience, in Cognitive Neuroscience of Emotion. Edited by Lane RD, Nadel L. Oxford, UK, Oxford University Press, 2000, pp 345-370Google Scholar