Functional Magnetic Resonance Imaging Evidence for Disrupted Basal Ganglia Function in Schizophrenia
Abstract
OBJECTIVE: This study was an examination of basal ganglia dysfunction in schizophrenia using functional magnetic resonance imaging (fMRI). METHOD: The authors used a motor sequencing task to investigate activation of the caudate, anterior putamen plus globus pallidus, and posterior putamen plus globus pallidus in eight subjects with schizophrenia and 12 group-matched comparison subjects. Differences in activation of the thalamus, the target of direct output from the globus pallidus, were also examined. RESULTS: The schizophrenia subjects showed significant bilateral deficits in the posterior putamen, globus pallidus, and thalamus but not the anterior putamen plus globus pallidus or caudate. Functional connectivity analysis revealed that the deficits in thalamic activation were related to deficits in posterior putamen and globus pallidus activation. CONCLUSIONS: These results provide fMRI evidence for basal ganglia dysfunction in subjects with schizophrenia and suggest that this deficit results in disrupted outflow to the thalamus. These deficits may underlie the behavioral impairments in goal-directed action observed in schizophrenia.
Motor abnormalities, such as catatonia, pacing, rocking, grimacing, posturing, and other stereotyped behavior, as well as avolition and general psychomotor poverty are common clinical symptoms of schizophrenia (1). Many of these “negative symptoms” of schizophrenia bear a striking similarity to symptoms commonly observed in basal ganglia disorders (2). Electrophysiological, lesion, and brain imaging studies (3–7) have shown that the basal ganglia play an essential role in adaptive behavior in general and movement initiation, control, and sequencing in particular. Taken together, these findings suggest that the movement abnormalities observed in schizophrenia may arise from basal ganglia dysfunction.
Positron emission tomography imaging studies have indicated abnormal resting-state metabolic rates in the caudate and putamen in subjects with schizophrenia. However, the results of these studies have been ambiguous, with some studies pointing to lower (8, 9) and others to higher (10) metabolic rates in subjects with schizophrenia. There have, however, been few investigations of basal ganglia dysfunction in schizophrenia with motor tasks that specifically activate the basal ganglia. Functional magnetic resonance imaging (fMRI) provides adequate spatial resolution to investigate deficits in specific basal ganglia nuclei, but its use has so far been limited because many motor tasks do not dependably activate the basal ganglia (11). In this study, we used a motor sequencing task, which we had previously shown to reliably and differentially activate the caudate, anterior putamen plus globus pallidus, and posterior putamen plus globus pallidus (11), to investigate basal ganglia function in schizophrenia. We hypothesized that subjects with schizophrenia would show significant deficits in the basal ganglia and that these deficits would result in reduced outflow into the thalamus. We performed a functional connectivity analysis (12) using analysis of covariance (ANCOVA) to investigate whether the observed thalamic deficits were related to deficits in outflow from the globus pallidus.
Method
Eight right-handed men who met the DSM-IV criteria for schizophrenia were matched as a group to 12 normal, healthy comparison subjects for gender, age, education, intelligence, parent/caregiver socioeconomic status, and handedness. The mean ages of the comparison subjects and patients with schizophrenia were 42.5 (SD=4.0) and 44.0 (SD=4.8), respectively. Their mean education levels were 14.5 years (SD=0.9) and 13.6 years (SD=1.5). As indexed by the National Adult Reading Test (13), their mean IQs were 113.9 (SD=7.5) and 108.3 (SD=10.0). The mean ratings of parent/caregiver socioeconomic status (14) were 2.7 (SD=0.6) and 3.0 (SD=1.0). Their mean scores for handedness (15) were 19.3 (SD=7.7) and 16.0 (SD=3.0), indicating that the patients with schizophrenia were slightly less right-handed than the comparison subjects. All of the subjects with schizophrenia had been receiving stable doses of medication for at least 2 weeks before the MRI scan. Written informed consent was obtained after complete description of the study.
The task consisted of 12 alternating 40-second epochs of passive fixation and arm movements as described in our earlier report (11). Briefly, the subject rested the closed fist of his right hand on the base of a palm-shaped keypad. He heard numbers between 1 and 4 every 2 seconds and made arm movements to corresponding “fingers” on the keypad.
Images were acquired on a 1.5-T GE scanner. During task performance, 12 axial slices (4.35≥4.35≥6.00 mm3), roughly extending –30 to 42 mm relative to the anterior commissure, were acquired by using a T2*-weighted gradient echo spiral pulse sequence; to aid in localization, high-resolution T1-weighted images were also acquired (11).
The fMRI data were analyzed by using SPM 96 (http://www. fil.ion.ucl.ac.uk/spm). The images were corrected for movement and normalized to stereotaxic coordinates. Time series regression analysis was performed on the individual subject data to determine brain activation during movement sequencing, compared to that during passive fixation. Voxel-wise t statistics were computed and normalized to z scores.
Analysis of variance (ANOVA) with group, region of interest, and hemisphere as factors was used to investigate group and regional differences in activation. The regions of interest included the caudate, the anterior putamen plus globus pallidus, the posterior putamen plus globus pallidus, and the thalamus. The anterior commissure separated the anterior and posterior putamen plus globus pallidus. The percentage of voxels activated above a threshold of z<2.33 (p<0.01) within each region of interest for each subject was used as the measure of activation. ANCOVA was used to explore group differences in functional connectivity. This analysis was used to investigate whether activation differences in one region (e.g., thalamus) were solely accounted for by activation differences observed in another region (e.g., posterior putamen plus globus pallidus).
Results
Head movement for the comparison subjects (mean=1.297 mm, SD=0.515) and subjects with schizophrenia (mean=1.667 mm, SD=0.906) was small and did not differ significantly between groups (t=–1.17, df=18, p=0.26; two-tailed).
An ANOVA of the interaction among group, region of interest (caudate, anterior putamen plus globus pallidus, posterior putamen plus globus pallidus) and hemisphere revealed a significant group-by-region interaction (F=3.42, df=2, 36, p=0.05, ε2=0.16). No other interactions or effects were significant. Follow-up analysis showed that the subjects with schizophrenia had significantly less activation than the comparison subjects in the posterior putamen plus globus pallidus (t=2.44, df=18, p=0.03, ε2=0.25) but not the anterior putamen plus globus pallidus (t=0.89, df=18, p=0.39, ε2=0.04) or caudate (t=0.16, df=18, p=0.88, ε2<0.01) (Figure 1).
Next, we examined the effects of input from the caudate on activation of the posterior putamen plus globus pallidus by using an ANCOVA model. Activation in the caudate was used as a covariate, and activation in the posterior putamen plus globus pallidus was the dependent variable, with group and hemisphere as factors. There was a significant main effect of group—the subjects with schizophrenia had significantly less activation in the posterior putamen plus globus pallidus (F=8.06, df=1, 16, p=0.02, ε2=0.34). There was no significant group-by-hemisphere interaction (F=0.49, df=1, 18, p=0.50, ε2=0.02) or main effect of hemisphere (F=0.02, df=1, 18, p=0.91, ε2<0.01). A similar pattern of results was observed when activation in the anterior putamen plus globus pallidus was used as the covariate and activation in the posterior putamen plus globus pallidus was the dependent variable. The subjects with schizophrenia showed deficits in the posterior putamen plus globus pallidus (F=7.63, df=1, 16, p=0.02, ε2=0.32) with no effect of hemisphere (F=0.02, df=1, 18, p=0.91, ε2<0.01) or group-by-hemisphere interaction (F=0.49, df=1, 18, p=0.50, ε2=0.02). These results suggest that the deficits in the posterior putamen plus globus pallidus arise locally rather than being driven by reduced activity upstream in the basal ganglia circuit.
We then examined activation in the thalamus, which is the main target site for outflow from the globus pallidus. The subjects with schizophrenia had significantly less activation (F=6.95, df=1, 18, p=0.02, ε2=0.28) than the comparison subjects, but the groups showed no effect of hemisphere (F=0.41, df=1, 18, p=0.53, ε2=0.02) or group-by-hemisphere interaction (F=0.92, df=1, 18, p=0.35, ε2=0.05).
Next, we examined the effects of input from the posterior putamen plus globus pallidus on thalamic activation. Activation of the posterior putamen plus globus pallidus was used as a covariate, and thalamic activation was the dependent variable, with group and hemisphere as factors. No significant group differences were observed in the thalamus (F=0.74, df=1, 16, p=0.41, ε2=0.04). There was also no significant group-by-hemisphere interaction (F=0.92, df=1, 18, p=0.35, ε2=0.05) or main effect of hemisphere (F=0.41, df=1, 18, p=0.53, ε2=0.02). This result suggests that deficits in the thalamus are driven by deficits in the posterior putamen plus globus pallidus.
Discussion
The movement-sequencing task used in the present study involved simple motor movements. Nevertheless, the subjects with schizophrenia showed significant deficits in basal ganglia activation. They had significantly less activation than the comparison subjects in the left and right posterior putamen plus globus pallidus but not the anterior putamen plus globus pallidus or caudate. The subjects with schizophrenia also showed significant deficits in the thalamus, the main target of output from the posterior putamen plus globus pallidus. These results suggest that outflow from the posterior basal ganglia is abnormal in people with schizophrenia. These deficits may underlie dysfunction in sensory-motor processing as well as the movement-related abnormalities commonly observed in subjects with schizophrenia.
The neural basis of the observed deficits was further investigated by modeling the input-output relations between the different regions of interest by using a multistage functional connectivity analysis. The analysis suggested that the deficits observed in the posterior putamen plus globus pallidus were not accounted for by differences in input from either the anterior putamen plus globus pallidus or caudate and that the posterior putamen plus globus pallidus is the primary locus of deficits in the basal ganglia. In contrast, thalamic deficits observed in subjects with schizophrenia result directly from deficits in the posterior putamen plus globus pallidus; that is, abnormal outflow from the basal ganglia–thalamic subsystem appears to originate in the posterior basal ganglia. The present study did not have adequate resolution to localize this abnormality more precisely, to the posterior putamen or internal or external segments of the globus pallidus. Since the thalamus receives direct projections from the globus pallidus, rather than the putamen, we hypothesize that the globus pallidus itself may be dysfunctional in schizophrenia. In the comparison subjects, activation was observed primarily in the ventral lateral and ventral anterior nuclei of the thalamus. These thalamic regions are thought to be part of the “motor loop” that projects to the supplementary motor area and presupplementary motor area (16). In the subjects with schizophrenia, these activations were significantly weaker and the precise voxels activated were more variable across subjects.
Our results provide what we believe to be the first fMRI evidence for disruption of basal ganglia–thalamic circuits in schizophrenia in the context of externally paced movement sequencing. Disruptions in the posterior basal ganglia and thalamus, in particular, may underlie a functional disconnection between networks involved in integrating sensory signals and action. Thus, although the prefrontal and temporal cortices (17) have been the main focus of pathology in schizophrenia, our data support the hypothesis (1, 18) that deficits in the basal ganglia–thalamic system may play an important role in the dysfunctional goal-directed behavior seen in subjects with schizophrenia. We further hypothesize that dysfunction in circuits involving thalamocortical circuits may be at least partly related to dysfunction in the basal ganglia.
Further studies are needed to investigate the relation between deficits in specific basal ganglia nuclei and behavioral measures such as reaction time and accuracy. The effects of reduced thalamic output on the supplementary motor area and presupplementary motor area, which play key roles in movement planning, also need to be examined.
Received July 18, 2000; revision received Oct. 17, 2000; accepted Nov. 8, 2000. From the Department of Psychiatry and Behavioral Sciences, Stanford University School of Medicine; and the Psychiatry Service, VA Palo Alto Health Care System, Palo Alto, Calif. Address reprint requests to Dr. Menon, Department of Psychiatry and Behavioral Sciences, Stanford University School of Medicine, Stanford, CA 94305-5719; [email protected] (e-mail). Supported by the Department of Veterans Affairs, grant AA-05965 from the National Institute on Alcohol Abuse and Alcoholism, grants MH-30854 and MH-58007 from NIMH, and a Young Investigator Award to Dr. Menon from the National Alliance for Research on Schizophrenia and Depression. The authors thank Bill Faustman for patient rating, Christopher Galloway for help with demographic data, Jennifer Johnson for assistance with scanning, and Sarah Rawson for help with subject recruitment.
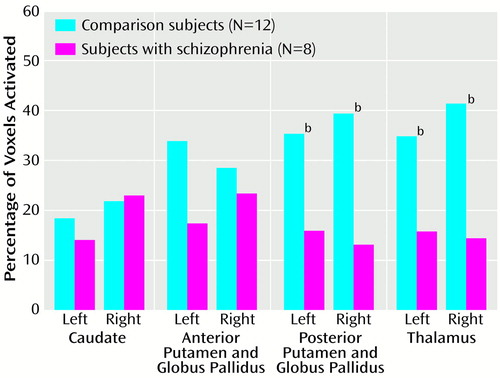
Figure 1. Activation of the Basal Ganglia and Thalamus During Performance of a Motor Sequencing Task by Subjects With Schizophrenia and Healthy Comparison Subjectsa
aActivation was defined as a difference between task performance and passive fixation that yielded a z score greater than 2.33 (p<0.01).
bSignificantly less activation among the subjects with schizophrenia than among the comparison subjects (two-tailed t test, p<0.05).
1. Andreasen NC: A unitary model of schizophrenia: Bleuler’s “fragmented phrene” as schizencephaly. Arch Gen Psychiatry 1999; 56:781–787Crossref, Medline, Google Scholar
2. Sandyk R, Kay SR: The relationship of negative schizophrenia to parkinsonism. Int J Neurosci 1990; 55:1–59Crossref, Medline, Google Scholar
3. Gardiner TW, Nelson RJ: Striatal neuronal activity during the initiation and execution of hand movements made in response to visual and vibratory cues. Exp Brain Res 1992; 92:15–26Crossref, Medline, Google Scholar
4. Kermadi I, Joseph JP: Activity in the caudate nucleus of monkey during spatial sequencing. J Neurophysiol 1995; 74:911–933Crossref, Medline, Google Scholar
5. Carli M, Evenden JL, Robbins TW: Depletion of unilateral striatal dopamine impairs initiation of contralateral actions and not sensory attention. Nature 1985; 313:679–682Crossref, Medline, Google Scholar
6. Schultz W, Romo R: Neuronal activity in the monkey striatum during the initiation of movements. Exp Brain Res 1988; 71:431–436Crossref, Medline, Google Scholar
7. Brooks DJ: The role of the basal ganglia in motor control: contributions from PET. J Neurol Sci 1995; 128:1–13Crossref, Medline, Google Scholar
8. Wolkin A, Jaeger J, Brodie JD, Wolf AP, Fowler J, Rotrosen J, Gomez-Mont F, Cancro R: Persistence of cerebral metabolic abnormalities in chronic schizophrenia as determined by positron emission tomography. Am J Psychiatry 1985; 142:564–571Link, Google Scholar
9. Buchsbaum MS, Hazlett EA: Positron emission tomography studies of abnormal glucose metabolism in schizophrenia. Schizophr Bull 1998; 24:343–364Crossref, Medline, Google Scholar
10. Biver F, Goldman S, Luxen A, Delvenne V, De Maertelaer V, De La Fuente J, Mendlewicz J, Lotstra F: Altered frontostriatal relationship in unmedicated schizophrenic patients. Psychiatry Res 1995; 61:161–171Crossref, Medline, Google Scholar
11. Menon V, Glover GH, Pfefferbaum A: Differential activation of dorsal basal ganglia during externally and self paced sequences of arm movements. Neuroreport 1998; 9:1567–1573Google Scholar
12. Fletcher P, McKenna PJ, Friston KJ, Frith CD, Dolan RJ: Abnormal cingulate modulation of fronto-temporal connectivity in schizophrenia. Neuroimage 1999; 9:337–342Crossref, Medline, Google Scholar
13. Nelson HE: The National Adult Reading Test (NART): Test Manual. Windsor, UK, NFER-Nelson, 1982Google Scholar
14. Hollingshead AB: Four-Factor Index of Social Status. New Haven, Conn, Yale University, Department of Sociology, 1975Google Scholar
15. Crovitz HF, Zener KA: Group test for assessing hand and eye dominance. Am J Psychol 1962; 75:271–276Crossref, Medline, Google Scholar
16. Alexander GE, Crutcher MD: Functional architecture of basal ganglia circuits: neural substrates of parallel processing. Trends Neurosci 1990; 13:266–271Crossref, Medline, Google Scholar
17. Goldman-Rakic PS, Selemon LD: Functional and anatomical aspects of prefrontal pathology in schizophrenia. Schizophr Bull 1997; 23:437–458Crossref, Medline, Google Scholar
18. Graybiel AM: The basal ganglia and cognitive pattern generators. Schizophr Bull 1997; 23:459–469Crossref, Medline, Google Scholar