Hippocampal Volume in Adolescent-Onset Alcohol Use Disorders
Abstract
OBJECTIVE: Alcohol use disorders (defined as DSM-IV alcohol dependence or abuse) are prevalent and serious problems among adolescents. As adolescence is marked by progressive hippocampal development, this brain region may be particularly susceptible to the adverse effects of adolescent alcohol use disorders. This study compared the hippocampal volumes of adolescents and young adults with adolescent-onset alcohol use disorders to those of healthy matched comparison subjects. METHOD: Magnetic resonance imaging was used to measure the hippocampal volumes and volumes of comparison brain regions in 12 subjects with alcohol use disorders and 24 comparison subjects matched on age, sex, and handedness. RESULTS: Both left and right hippocampal volumes were significantly smaller in subjects with alcohol use disorders than in comparison subjects. Total hippocampal volume correlated positively with the age at onset and negatively with the duration of the alcohol use disorder. Intracranial, cerebral, and cortical gray and white matter volumes and measures of the mid-sagittal area of the corpus callosum did not differ between groups. CONCLUSIONS: In the mature brain, chronic alcohol use disorders are associated with graded global brain dysmorphology. Although the etiology, neuropsychological consequences, and permanence of these hippocampal findings need to be further examined, these findings suggest that, during adolescence, the hippocampus may be particularly susceptible to the adverse effects of alcohol.
Alcohol use disorders (defined as DSM-IV alcohol dependence or abuse) are prevalent and serious problems among adolescents. In the late adolescent developmental period, approximately 10% of adolescents meet diagnostic criteria for an alcohol use disorder (1–3). While the psychosocial consequences of adolescent alcohol use disorders have been explored (4, 5), there has been little study of the neurobiological effects of alcohol during this developmental period. In the mature brain, the CNS abnormalities that result from chronic alcohol use disorders are characterized by a continuum of graded brain dysmorphology, ranging from more subtle clinical pathology to classic alcohol-related disorders (6, 7). However, the effects of alcohol on the actively developing adolescent brain may differ from the effects on the adult brain.
Progressive and regressive processes of brain development mark adolescence. The hippocampus and prefrontal cortex are developing more actively in adolescence than in adulthood (for review see references 8–10). Subcortical gray matter and limbic system structures (septal area, hippocampus, amygdala) show an increase in volume. At the same time, cortical structures such as the prefrontal cortex actually decrease in volume, perhaps as a result of synaptic pruning processes, until the third decade (11). As the brain is developing more actively during adolescence, the effects of an alcohol use disorder during adolescence may be different from those seen in adulthood.
To our knowledge, there are no published neuroimaging studies to date on the neurobiological consequences of excessive drinking in adolescence. In this study, we examined the hippocampus, amygdala, and other comparison brain regions of adolescents and young adults with an adolescent-onset alcohol use disorder and matched healthy comparison subjects. We hypothesized that hippocampal and amygdaloid volumes would be smaller in subjects with alcohol use disorders than in the healthy comparison subjects. The neurotoxic effects of alcohol on adult global brain morphology (intracranial volume, cortical gray and white matter, lateral ventricles, and corpus callosum) are known to be age related (12). Thus, we hypothesized that with this cohort, global brain morphology would not differ between the two groups.
Method
Subjects
Twelve adolescents and young adults with an adolescent-onset alcohol use disorder (five male and seven female subjects; mean age=17.2 years [SD=2.2]) and 24 healthy comparison subjects (10 male and 14 female subjects; mean age=17.0 years [SD=2.4]) were recruited (age range for all subjects=13.5–21.0 years). Because of the high degree of known variability in volume of brain structures (9, 13), each subject with an alcohol use disorder was case matched with two healthy comparison subjects for age (within 6 months; z=0.1, df=1, p=0.92) as well as for sex and handedness. Subjects with alcohol use disorders were similar to the comparison subjects with respect to height (mean=170.0 cm [SD=11.7] and 169.3 cm [SD=9.9], respectively; t=0.17, df=34, p=0.87); weight (mean=68.3 kg [SD=11.1] and 71.1 kg [SD=17.5]; t=0.50, df=34, p=0.62); and full-scale IQ (mean=109.0 [SD=10.5] and 110.5 [SD=17.6]; t=0.28, df=34, p=0.78). The socioeconomic status of the two groups also did not differ (mean=34.4 [SD=6.3] and 38.5 [SD=9.9]; t=1.29, df=34, p=0.20), with the mean scores indicating that both groups were on average from working class families. The comparison group was recruited by advertisement from the community and had no lifetime histories of psychiatric disorders, including alcohol and substance use disorders.
Substance use disorder diagnoses were determined by using a modified form of the Structured Clinical Interview for DSM-IV (see reference 14 for details). Information regarding alcohol- and drug-related areas was gathered by means of direct interviews, since family informants typically underreport alcohol and drug involvement (15).
Other axis I mental disorders were assessed by using a modified version of the Schedule for Affective Disorders and Schizophrenia for School-Age Children (K-SADS) (16) and the K-SADS Epidemiologic Version (K-SADS-E) (17) interviews, with both adolescent and parent(s) as informants. An expanded assessment of posttraumatic stress disorder (PTSD) was completed as part of the K-SADS Present and Lifetime Version (K-SADS-PL) (18).
Consensus diagnoses were reached among the interviewer, assessment coordinator, and a clinically experienced faculty psychiatrist or psychologist by using the best-estimate method (19–21). A date of onset, defined as the time at which diagnostic criteria were first met, was determined for each disorder (22). The alcohol use disorders group consisted of seven subjects with lifetime alcohol dependence and five subjects with lifetime alcohol abuse. Comorbid conditions included other substance abuse or dependence (cannabis [N=9] or hallucinogens [N=2]), major depressive disorder (N=9), conduct disorder (N=5), PTSD (N=5), attention deficit hyperactivity disorder (ADHD) (N=5), oppositional defiant disorder (N=2), generalized anxiety disorder (N=2), and bipolar disorder (N=1). The mean age at onset for an alcohol use disorder was 15.9 years (SD=2.4). While the mean age at onset for the nine subjects with cannabis abuse was 15.3 years (SD=2.3), for seven of these subjects the alcohol use disorder diagnosis preceded their cannabis use disorder diagnosis. Brain structural volumes for four of the subjects with alcohol use disorders (with comorbid PTSD) and 10 of the healthy comparison subjects were previously reported (8).
Subjects were excluded from the study if the following were found: use of drugs or alcohol during the 2 weeks before the magnetic resonance imaging (MRI) scan, assessed by a urine drug screen and alcohol breathalyzer test within 12 hours of the scan; presence of a clinically significant medical or neurological illness; gross obesity (weight greater than 150% of ideal body weight) or growth failure (height under third percentile); full-scale IQ below 80; pregnancy; or inability to consent to the protocol because of insufficient English skills. After a complete description of the study was given to the subject and parents if appropriate, written informed consent was obtained. Subjects received monetary compensation for participation.
MRI Acquisition
MRI scans were performed at the University of Pittsburgh Medical Center Magnetic Resonance Research Center by using a Signa 1.5-T system (GE Medical Systems, Milwaukee). A sagittal scout series verified subject position, cooperation, and image quality. A three-dimensional, spoiled gradient recalled acquisition in the steady state pulse sequence was used to obtain 124 contiguous images with slice thickness of 1.5 mm in the coronal plane (TE=5 msec, TR=25 msec, flip angle=40˚, acquisition matrix=256 ¥ 192, number of excitations=1, field of vision=24 cm). Coronal sections were obtained perpendicular to the anterior commissure/posterior commissure line to provide a more reproducible guide for image orientation. Axial proton density and T2-weighted images were obtained to enable exclusion of structural abnormalities on the MRI scan. A neuroradiologist reviewed all scans and ruled out clinically significant abnormalities. All subjects tolerated the procedure well. No sedation was used.
Image Analysis
The imaging data were transferred from the MRI unit to a computer workstation and analyzed using the IMAGE software (version 1.52) developed at the National Institutes of Health (23), which provides valid and reliable volume measurements of specific structures by using a semiautomated segmentation approach. All measurements were made by trained and reliable raters who were blind to subject information. Independent designation of regions for all structures described had interrater reliabilities that ranged from 0.91 (corpus callosum regions) to 0.99 (intracranial volume) and intrarater reliabilities that ranged from 0.97 (corpus callosum regions, amygdala and hippocampal volumes) to 0.99 (intracranial volume). Complete methodological details have been previously described (8) and are only briefly presented here.
Intracranial volumes were calculated by summing areas of successive coronal slices, including gray and white matter and CSF volumes, and multiplying by slice thickness. Cerebral volumes were measured in the same manner after exclusion of cerebellum and brainstem.
Total cerebral gray and white matter volumes were calculated by using a semiautomated segmentation algorithm. This computerized segmentation technique uses mathematically derived cutoffs for gray matter-white matter CSF partitions with histograms of signal intensities.
The amygdala and hippocampus were manually traced in the coronal plane. Then the areas were summed for each slice and multiplied by slice thickness, using the most anterior slice in which the temporal stem was first visible. The most anterior slice showing the mammillary bodies was used as the amygdala-hippocampal boundary. The ambient cistern defined the medial border of the anterior hippocampus. The superior border of the hippocampus was bounded anteriorly by the temporal horn and posteriorly by the fornix. Our measurement of the hippocampal formation included the cornu ammonis, dentate gyrus, and subiculum.
Right and left lateral ventricle measurements were obtained using a manual tracing technique in the coronal plane. Measures were summed for each slice and multiplied by slice thickness. This technique was used rather than measurements of CSF to reliably exclude the choroid plexus.
The corpus callosum was identified from a single midsagittal section selected as the slice showing full visualization of the anterior and posterior commissures and the cerebral aqueduct (8). This area was divided into seven regions (rostrum, genu, rostral body, anterior and posterior midbody, isthmus, and splenium).
Statistical Methods
Data distributions were examined for normality using Shapiro and Wilks’s W statistic. Where significantly nonnormal distributions were found (amygdala and lateral ventricle volumes), the data were log transformed to normalize the distributions before applying parametric tests. In cases where no transformation normalized the data (age), nonparametric tests were used. Categorical demographic variables were compared using t tests or Fisher’s exact tests as appropriate. Between-group differences were tested by means of analysis of variance after controlling for intracranial volume. In testing for covariate effects, such as sex and interactions (sex by group), multivariate regression analysis was used. Brain structures for which adjusted least squares means differed significantly between the groups (total hippocampus) were correlated with clinical data by using Spearman correlation coefficients. All significance tests involving the main hypothesis were two-tailed, with alpha=0.05. A reduced critical value of p<0.01 was used in our exploratory analyses of sex, sex-by-group, and clinical correlation data to control for type I errors.
Results
Brain Measurements
Subjects with alcohol use disorders had smaller left and right hippocampal volumes than did the healthy comparison subjects (Figure 1 and Figure 2). Intracranial and cerebral volumes, cortical gray and white matter, right and left amygdala volumes, and corpus callosum area did not differ between groups (Table 1). Corpus callosum regional areas, which reflect axons from specific cortical regions, also did not differ between groups. Hippocampal volumes remained smaller even after adjustment for intracranial volume, sex, and the sex-by-group interaction (F=6.47, df=1, 31, p<0.02). Significantly lower hippocampal volumes were seen in subjects with alcohol use disorders with PTSD (mean=7.64 cm3, SD=0.75) than in their matched healthy comparison subjects (mean=9.03 cm3, SD=1.34) (F=4.63, df=1, 13, p=0.05); a suggestive but nonsignificant difference was seen between subjects with alcohol use disorders without PTSD (mean=8.43 cm3, SD=0.46) and their matched healthy comparison subjects (mean=9.01 cm3, SD=0.83) (F=4.18, df=1, 19, p<0.06).
Relationships Between Hippocampal Volume and Demographic and Clinical Factors
Total hippocampal volumes adjusted for intracranial volumes correlated positively with the age at onset (rs=0.78, N=12, p=0.004) and negatively with the duration in years (rs=–0.73, N=12, p<0.007) of the alcohol use disorder. There were no significant correlations between adjusted hippocampal volumes and alcohol consumption (average drinks per month) in the 30 days before clinical assessment (rs=0.45, N=12, p=0.14). There were no significant correlations between adjusted hippocampal volumes and the age at onset (rs=0.54, N=9, p=0.13) or duration (rs=–0.17, N=9, p=0.69) of a comorbid cannabis use disorder.
Overall, male subjects had larger intracranial volumes than did female subjects (F=9.20, df=1, 32, p=0.005); there were no significant sex-by-group effects.
Discussion
Adolescents and young adults with adolescent-onset alcohol use disorders were found to have significantly smaller left and right hippocampal volumes than healthy matched comparison subjects. Intracranial, cerebral, cortical gray and white matter volumes, right and left amygdala volumes, and total corpus callosum area and its comparison regional measures did not differ between groups. Hippocampal measures adjusted for intracranial volumes correlated positively with the age at onset and negatively with the duration in years of the alcohol use disorder. There were no significant correlations between hippocampal measures and alcohol consumption. To our knowledge, this is the first study to examine the association of an adolescent-onset alcohol use disorder with the brain morphology of adolescents and young adults.
There are several possible explanations for the finding of decreased hippocampal volumes in adolescents and young adults with adolescent-onset alcohol use disorders. Alcohol-related neurotoxic effects on the hippocampus may be mediated by N-methyl-d-aspartate (NMDA) receptors, a class of excitatory amino acid receptors, or by the neurotrophic functions of NMDA receptors, thus causing disrupted hippocampal development in adolescents. A smaller hippocampus also may indicate the presence of another risk variable common to alcohol use disorders and smaller hippocampal size (i.e., comorbidity). Finally, a preexisting smaller hippocampus may itself be a risk factor for an adolescent-onset alcohol use disorder.
Smaller hippocampal volumes in adults with alcohol use disorders than in adults without alcohol use disorders have been reported (24–26). In animals, hippocampal damage is seen with chronic alcohol administration (27, 28). Alcohol may have direct toxic effects on the hippocampus through NMDA receptors that are independent of seizure activity (29). Findings from animal studies showed that the hippocampus exhibits enhanced NMDA-mediated neurotoxicity during alcohol withdrawal (30, 31). Chronic alcohol consumption leads to up-regulation of NMDA receptors (32). Alcohol withdrawal leads to increased excitatory transmission. This process can result in discrete excitotoxic neuronal damage due to repetitive, excessive stimulation of NMDA receptors (33, 34). Furthermore, chronic intermittent alcohol exposure in rats results in hippocampal cell loss, an effect not seen in animals constantly exposed to alcohol (35, 36). Adolescents with alcohol use disorders tend to binge drink (37). Since hippocampus maturation continues during adolescence and young adulthood, the pattern of adolescent drinking may interact with aspects of hippocampal development. Alternatively, inhibition of NMDA receptors by ethanol may result in a complex disinhibition of several excitatory pathways that lead to a neuron-necrotizing reaction of cortical and limbic structures (38). This latter toxic mechanism is age dependent and becomes fully sensitive between puberty and adulthood (39). Furthermore, NMDA receptors are critically involved in the process of activity-dependent synaptic elimination in the adolescent brain (40). This plasticity declines during adolescence in parallel with reduction in NMDA receptor activity. Hence, disrupted hippocampal development may be the result of reduced NMDA receptor activity and, consequently, a disruption of its neurotrophic functions. Thus, the adolescent hippocampus may be particularly vulnerable to the effects of alcohol through NMDA-mediated mechanisms.
High comorbidity is typical with adolescent-onset alcohol use disorders (22). As in our cohort, other studies have shown that a substantial percentage of adolescents with alcohol use disorders have other comorbid substance use disorders (particularly cannabis abuse) as well as conduct disorder, ADHD, mood disorders, and PTSD (22). Our subjects with alcohol use disorders had high rates of comorbid major depression. Smaller hippocampal volumes were reported in clinical conditions thought to be associated with increased corticosteroid levels. A substantial proportion of adult major depressive disorder patients exhibit cortisol hypersecretion (for review see reference 41). However, cortisol hypersecretion is not strongly associated with either major depressive disorder or dysthymia in children and adolescents (42). One study showed that depressed prepubertal children had lower cortisol secretion during the first 4 hours after sleep onset with no overall 24-hour differences between depressed subjects and a comparison group (43). Decreased hippocampal volumes have not been consistently shown in adults with major depression (44, 45), but a recent study found decreased hippocampal volumes in adult women with recurrent major depression (46). These findings correlated with longer duration of depressive illness and were not associated with substance abuse. No sex-by-group effects were seen in hippocampal volumes in our subjects with alcohol use disorders.
Smaller hippocampal volumes were reported in adults with Cushing’s syndrome (47) and conditions of traumatic stress such as combat veterans with PTSD (48, 49), adult PTSD related to childhood abuse (50), and female adult survivors of childhood sexual abuse (51). Among adolescents with alcohol use disorders, PTSD is typically preceded by a history of physical or sexual abuse (22, 52–54). In the adult PTSD studies, the incident rates of comorbid lifetime diagnoses of alcohol abuse and dependence were high, ranging from 71% to 76%. These investigators controlled for lifetime alcohol consumption by including subjects in their comparison group with alcohol use disorders and using standardized lifetime alcohol use interviews. However, in a cross-sectional investigation, we showed by means of MRI scans that maltreated children and adolescents with PTSD (N=43) had smaller brain structural measures of intracranial volumes, midsagittal areas of the corpus callosum, and larger ventricular volumes than nonmaltreated healthy comparison subjects (N=61) (8). The predicted decrease in hippocampal volume was not seen in these young PTSD subjects. In these adult PTSD studies and our child PTSD study (8), maltreated subjects with PTSD exhibited high degrees of comorbidity, particularly for mood disorders. For example, 36 (83.7%) of 43 child and adolescent PTSD subjects had a depressive disorder. However, maltreated children and adolescents with PTSD did have less comorbid histories of alcohol and substance abuse, as only four of our adolescent subjects had this history (8); they were included in this present study. In the present study, we found a suggestive although nonsignificant decrease in hippocampal volume in the alcohol use disorders subgroup without PTSD, when compared with their matched comparison subjects. Ethanol has been shown to stimulate pituitary corticotrophs resulting in hypercortisolemia, a feature of heavy drinking (55, 56). The hippocampus is also rich in glucocorticoid receptors, and a high level of cortisol is damaging to the hippocampus (57). Comorbid adolescent-onset alcohol use disorders may contribute to some of the differences in hippocampal findings between children and adolescents and adults with PTSD. Alternatively, an interaction effect of an adolescent-onset alcohol use disorder and PTSD may be especially toxic to the hippocampus.
In MRI studies of children and adolescents with ADHD, no hippocampal differences were seen between those with ADHD and healthy comparison subjects (58). No hippocampal differences were seen between healthy matched comparison subjects and child and adolescent subjects who had generalized anxiety disorder without comorbid PTSD or a substance use disorder (59). To date, there are no published MRI hippocampal studies on adolescent conduct disorder or cannabis dependence. Cannabis dependence may be associated with 9-tetrahydrocannabinol-induced cell death in preclinical studies (for review see reference 60) and is a potential confound in our study. Smaller hippocampal volumes are seen in childhood-onset schizophrenia (61) and pediatric seizure disorders (62, 63). However, subjects with a history of psychosis were excluded from our study. Seizures and nutritional deficiencies may account for findings of decreased white matter and hippocampal volumes in alcohol-induced brain damage in adult alcohol use disorders. However, nutritional deficiencies and alcohol withdrawal seizures are very rare in adolescents (19) and were not seen in our subjects with alcohol use disorders.
Thus, hippocampal toxicity in adolescent-onset alcohol use disorders may be mediated by several mechanisms. Since we found that total hippocampal volume correlated positively with the age at onset and duration of an alcohol use disorder diagnosis, one may wonder if adolescence is a more vulnerable period for hippocampal toxicity. Still, the small cohort and effect sizes and extensive comorbidity in our subjects with alcohol use disorders may have contributed to these findings. Furthermore, preexisting smaller hippocampal volumes may be a risk factor for an adolescent-onset alcohol use disorder, regardless of comorbidity. Our preliminary findings suggest, but do not prove, that the adolescent hippocampus may be particularly susceptible to the effects of alcohol. However, this study did not have adequate power to examine comorbidity within subjects with alcohol use disorders, and further study of these issues is warranted.
Lezak (64) and Welsh and Pennington (65) have described the correlation between the hippocampus and the integrity of various memory functions that include storage and recall, rote learning, memory for verbal material with context, and spatial memory. In this study, we did not examine hippocampal function, so we do not know if our findings have clinical sequelae. Neurotoxic effects to the brain from chronic excessive drinking may be reversible (12, 66–68). This recovery process may be especially important for adolescent brain development, since adolescents with an alcohol use disorder who become sober may have a better chance for recovery of function than in adulthood because of the developmental plasticity of this time period.
In summary, unlike in the mature brain, where chronic alcohol use disorders are characterized by a continuum of graded brain dysmorphology, our preliminary findings suggest that the adolescent hippocampus may be particularly susceptible to the effects of alcohol. The etiology, neuropsychological consequences, and permanence of these changes need to be examined. Future MRI studies of individuals with an adolescent-onset alcohol use disorder as well as of at-risk adolescents are warranted.
![]() |
Presented in part at the 46th annual meeting of the American Academy of Child and Adolescent Psychiatry, Chicago, Oct. 19–24, 1999, and at the 38th annual meeting of the American College of Neuropsychopharmacology, Acapulco, Mexico, Dec. 12–16, 1999. Received Aug. 16, 1999; revision received Nov. 22, 1999; accepted Nov. 30, 1999. From the University of Pittsburgh Medical Center, Developmental Traumatology Neuroimaging Laboratory, and the Adolescent Alcohol Research Center, Western Psychiatric Institute and Clinic. Address reprint requests to Dr. De Bellis, University of Pittsburgh Medical Center, Developmental Traumatology Neuroimaging Laboratory, Western Psychiatric Institute and Clinic, 3811 O’Hara St., Pittsburgh, PA 15213; [email protected] (e-mail). Supported in part by NIMH grants to Dr. De Bellis (MH-O1324) and Dr. Keshavan (MH-01180 and MH-43687) and National Institute on Alcohol Abuse and Alcoholism grants AA-08746 and AA-00291 to Dr. Clark. The authors thank Grace Moritz, M.S.W., Cara Renzelli, M.S.Ed., Karin Frustaci, B.S., Jay N. Giedd, M.D., and Neal D. Ryan, M.D., for their assistance in this work and Kevin Lynch, Ph.D., for statistical consultation.
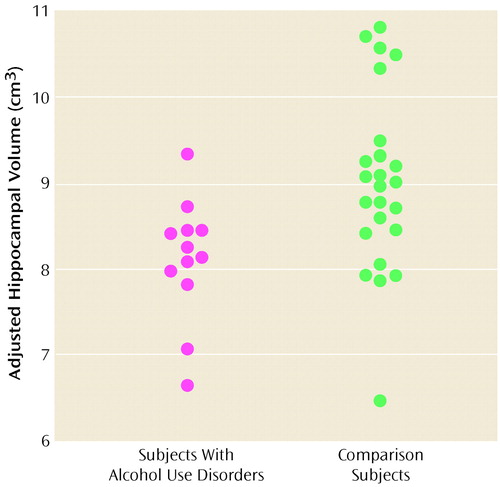
Figure 1. Hippocampal Volumes, Adjusted for Intracranial Volume, of Subjects With Adolescent-Onset Alcohol Use Disorders and Healthy Matched Comparison Subjectsa
aHippocampal volumes in subjects with alcohol use disorders (mean=8.10 cm3, SD= 0.70) were significantly lower than those of healthy comparison subjects (mean=9.02 cm3, SD=1.05) (F=7.11, df=1, 33, p=0.01).
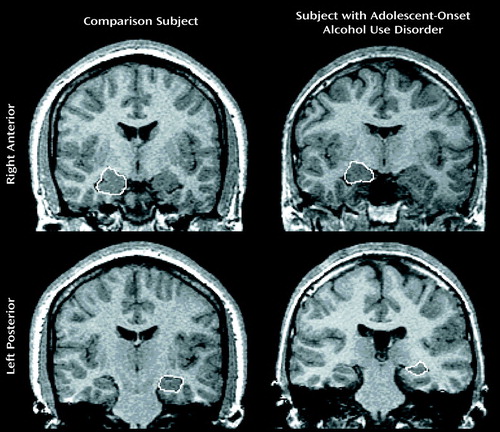
Figure 2. MRI Scans Depicting the Right Anterior and Left Posterior Hippocampus in a Subject With an Adolescent-Onset Alcohol Use Disorder and a Healthy Comparison Subject Matched for Age, Sex, and Handedness
1. Rohde P, Lewinsohn PM, Seeley JR: Psychiatric comorbidity with problematic alcohol use in high school students. J Am Acad Child Adolesc Psychiatry 1996; 35:101–109Crossref, Medline, Google Scholar
2. Reinhertz HA, Giaconia IM, Lefkowitz ES, Pakiz B, Frost AK: Prevalence of psychiatric disorders in a community population of older adolescents. J Am Acad Child Adolesc Psychiatry 1993; 32:369–377Crossref, Medline, Google Scholar
3. Cohen P, Cohen J, Kasen S, Velez CN, Hartmark C, Johnson J, Rojas M, Brook J, Streuning EL: An epidemiological study of disorders in late childhood and adolescence, I: age- and gender-specific prevalence. J Child Psychol Psychiatry 1993; 34:851–867Crossref, Medline, Google Scholar
4. Clark DB, Kirisci L: PTSD, depression, and adolescent alcohol use disorders and quality of life in adolescents. Anxiety 1996; 2:226–233Crossref, Medline, Google Scholar
5. Duncan SC, Alpert A, Duncan TE, Hops H: Adolescent alcohol use development and young adult outcomes. Drug Alcohol Depend 1997; 49:39–48Crossref, Medline, Google Scholar
6. Pfefferbaum A, Lim KO, Desmond JE, Sullivan EV: Thinning of the corpus callosum in older alcoholic men: a magnetic resonance imaging study. Alcohol Clin Exp Res 1996; 20:752–757Crossref, Medline, Google Scholar
7. Ragan PW, Singleton CK, Martin PR: Brain injury associated with chronic alcoholism. CNS Spectrums 1999; 4:66–87Google Scholar
8. De Bellis MD, Keshavan MS, Clark DB, Casey BJ, Giedd J, Boring AM, Frustaci K, Ryan ND: AE Bennett Research Award: developmental traumatology, part II: brain development. Biol Psychiatry 1999; 45:1271–1284Google Scholar
9. Giedd JN, Vaituzis AC, Hamburger SD, Lange N, Rajapakse JC, Kaysen D, Vauss YC, Rapoport JL: Quantitative MRI of the temporal lobe, amygdala, and hippocampus in normal human development: ages 4–18. J Comp Neurol 1996; 366:223–230Crossref, Medline, Google Scholar
10. Pfefferbaum A, Mathalon DH, Sullivan EV, Rawles JM, Zipursky RB, Lim KO: A quantitative magnetic resonance imaging study of changes in brain morphology from infancy to late adulthood. Arch Neurol 1994; 34:71–75Google Scholar
11. Jernigan TL, Sowell ER (eds): Magnetic Resonance Imaging Studies of the Developing Brain. Cambridge, UK, Cambridge University Press, 1997Google Scholar
12. Pfefferbaum A, Sullivan EV, Rosenbloom MJ, Mathalon DH, Lim KO: A controlled study of cortical gray matter and ventricular changes in alcoholic men over a 5-year interval. Arch Gen Psychiatry 1998; 55:905–912Crossref, Medline, Google Scholar
13. Giedd JN, Snell JW, Lange N, Rajapakse JC, Kaysen D, Vaituzis AC, Vauss YC, Hamburger SD, Kouch PL, Rapoport JL: Quantitative magnetic resonance imaging of human brain development: ages 4–18. Cereb Cortex 1996; 6:551–560Crossref, Medline, Google Scholar
14. Martin CS, Kaczunski N, Maisto S, Bukstein O, Moss H: Patterns of DSM-IV alcohol abuse and dependence symptoms in adolescent drinkers. J Stud Alcohol 1995; 56:672–680Crossref, Medline, Google Scholar
15. Kosten TA, Anton SF, Rounsaville BJ: Ascertaining psychiatric diagnoses with the family history method in a substance abuse population. J Psychiatr Res 1992; 26:135–147Crossref, Medline, Google Scholar
16. Chambers WJ, Puig-Antich J, Hirsch M, Paez P, Ambrosini PJ, Tabrazi MA, Davies M: The assessment of affective disorders in children and adolescents by semistructured interview: test-retest reliability of the Schedule for Affective Disorders and Schizophrenia for School-Age Children, Present Episode Version. Arch Gen Psychiatry 1985; 42:696–702Crossref, Medline, Google Scholar
17. Orvaschel H, Puig-Antich J: Schedule for Affective Disorders and Schizophrenia for School-Age Children—Epidemiologic Version (K-SADS-E), 4th revision. Pittsburgh, Western Psychiatric Institute and Clinic, 1987Google Scholar
18. Kaufman J, Birmaher B, Brent D, Rao U, Flynn C, Moreci P, Williamson D, Ryan N: Schedule for Affective Disorders and Schizophrenia for School-Age Children— Present and Lifetime Version (K-SADS-PL): initial reliability and validity data. J Am Acad Child Adolesc Psychiatry 1997; 36:980–988Crossref, Medline, Google Scholar
19. Clark DB: Psychiatric assessment, in Sourcebook on Substance Abuse: Etiology, Epidemiology, Assessment, and Treatment. Edited by Ott PJ, Tarter RE, Ammerman RT. Needham Heights, Mass, Allyn & Bacon, 1999, pp 197–211Google Scholar
20. Kosten TA, Rounsaville BJ: Sensitivity of psychiatric diagnosis based on the best estimate procedure. Am J Psychiatry 1992; 149:1225–1227Google Scholar
21. Leckman JF, Sholomskas D, Thompson WD, Belanger A, Weissman MM: Best estimate of lifetime psychiatric diagnosis: a methodological study. Arch Gen Psychiatry 1982; 39:879–883Crossref, Medline, Google Scholar
22. Clark DB, Pollock N, Bukstein OG, Mezzich AC, Bromberger JT, Donovan JE: Gender and comorbid psychopathology in adolescents with alcohol dependence. J Am Acad Child Adolesc Psychiatry 1997; 36:1195–1203Google Scholar
23. Rasband W: NIH IMAGE Manual. Bethesda, Md, National Institutes of Health, 1996Google Scholar
24. Jernigan TL, Butters N, DiTraglia G, Schafer K, Smith T, Irwin M, Grant I, Schuckit M, Cermak LS: Reduced cerebral grey matter observed in alcoholics using magnetic resonance imaging. Alcohol Clin Exp Res 1991; 15:418–427Crossref, Medline, Google Scholar
25. Badsberg-Jensen G, Pakkenberg B: Effect of chronic alcoholism on the human hippocampus. Histol Histopathol 1990; 5:349–357Medline, Google Scholar
26. Sullivan EV, Marsh L, Mathalon DH, Lim KO, Pfefferbaum A: Anterior hippocampal volume deficits in nonamnesic, aging chronic alcoholics. Alcohol Clin Exp Res 1995; 19:110–122Crossref, Medline, Google Scholar
27. Walker DW, King MA, Hunter BE: Alterations in the structure of the hippocampus after long-term ethanol consumption, in Alcohol-Induced Brain Damage, vol 22. Edited by Hunt VA, Nixon SJ. Washington, DC, US Department of Health and Human Services, 1993, pp 231–247Google Scholar
28. Lescaudron L, Jaffard R, Verna A: Modifications in the number and morphology of dendritic spines resulting from chronic ethanol consumption and withdrawal: a Golgi study in the mouse anterior and posterior hippocampus. Exp Neurol 1989; 106:156–163Crossref, Medline, Google Scholar
29. Lovinger DM, White G, Weight FF: Ethanol inhibits NMDA-activated ion current in hippocampal neurons. Science 1989; 243:1721–1724Google Scholar
30. Davidson M, Wilce P, Shanley BC: Increased sensitivity of the hippocampus in ethanol-dependent rats to toxic effect of N-methyl-D-aspartic acid in vivo. Brain Res 1993; 606:5–9Crossref, Medline, Google Scholar
31. Davidson M, Shanley B, Wilce P: Increased NMDA-induced excitability during ethanol withdrawal: a behavioral and histologic study. Brain Res 1995; 674:91–96Crossref, Medline, Google Scholar
32. Breese CR, Freedman R, Leonard SS: Glutamate receptor subtype expression in human postmortem brain tissue from schizophrenics and alcohol abusers. Brain Res 1995; 674:82–90Crossref, Medline, Google Scholar
33. Tsai G, Coyle JT: The role of glutamatergic neurotransmission in the pathophysiology of alcoholism. Annu Rev Med 1998; 49:173–184Crossref, Medline, Google Scholar
34. Becker HC: Alcohol withdrawal: neuroadaptation and sensitization. CNS Spectrums 1999; 4:38–65Medline, Google Scholar
35. Lundqvist C, Volk B, Knoth R, Alling C: Long-term effects of intermittent versus continuous ethanol exposure on hippocampal synapses of the rat. Acta Neuropathol 1994; 87:242–249Crossref, Medline, Google Scholar
36. Lundqvist C, Alling C, Knoth R, Volk B: Intermittent ethanol exposure of adult rats: hippocampal cell loss after one month of treatment. Alcohol Clin Exp Res 1995; 30:737–748Google Scholar
37. Deas D, Riggs P, Langenbucher J, Goldman M, Brown S: Adolescents are not adults: developmental considerations in alcohol users. Alcohol Clin Exp Res 2000; 24:232–237Crossref, Medline, Google Scholar
38. Olney JW, Farber NB: NMDA antagonists as neurotherapeutic drugs, psychotogens, neurotoxins, and research tools for studying schizophrenia. Neuropsychopharmacology 1995; 13:335–345Crossref, Medline, Google Scholar
39. Farber NB, Wozniak DF, Price MT, Labruyere J, Huss J, St Peter H, Olney JW: Age-specific neurotoxicity in the rat associated with NMDA receptor blockage: potential relevance to schizophrenia? Biol Psychiatry 1995; 38:778–796Google Scholar
40. Hofer M, Constantine-Paton M: Regulation of N-methyl-d-aspartate (NMDA) receptor function during the rearrangement of developing neuronal connections. Prog Brain Res 1994; 102:277–285Crossref, Medline, Google Scholar
41. De Bellis MD, Chrousos GP, Dorn LD, Burke L, Helmers K, Kling MA, Trickett PK, Putnam FW: Hypothalamic-pituitary-adrenal axis dysregulation in sexually abused girls. J Clin Endocrinol Metab 1994; 78:249–255Medline, Google Scholar
42. Casat CD, Powell K: The dexamethasone suppression test in children and adolescents with major depressive disorder: a review. J Clin Psychiatry 1988; 49:390–393Medline, Google Scholar
43. De Bellis MD, Dahl RE, Perel J, Birmaher B, Al-Shabbout M, Williamson DE, Nelson B, Ryan ND: Nocturnal ACTH, cortisol, growth hormone, and prolactin secretion in prepubertal depression. J Am Acad Child Adolesc Psychiatry 1996; 35:1130– 1138Google Scholar
44. Axelson DA, Doraiswamy PM, McDonald WM, Boyko OB, Tupler LA, Patterson L, Nemeroff CB, Ellinwood EJ, Krishnan KR: Hypercortisolemia and hippocampal changes in depression. Psychiatry Res 1993; 47:163–173Crossref, Medline, Google Scholar
45. Coffey CE, Wilkinson WE, Weiner RD, Parashos IA, Djang WT, Webb MC, Figiel GS, Spritzer CE: Quantitative cerebral anatomy in depression: a controlled magnetic resonance imaging study. Arch Gen Psychiatry 1993; 50:7–16Crossref, Medline, Google Scholar
46. Sheline YI, Sanghavi M, Mintun MA, Gado MH: Depression duration but not age predicts hippocampal volume loss in medically healthy women with recurrent major depression. J Neurosci 1999; 19:5034–5043Google Scholar
47. Starkman MN, Gebarksi SS, Berent S, Schteingart DE: Hippocampal formation volume, memory dysfunction, and cortisol levels in patients with Cushing’s syndrome. Biol Psychiatry 1992; 32:756–765Crossref, Medline, Google Scholar
48. Bremner JD, Randall P, Scott TM, Bronen RA, Seibyl JP, Southwick SM, Delaney RC, McCarthy G, Charney DS, Innis RB: MRI-based measurement of hippocampal volume in patients with combat-related posttraumatic stress disorder. Am J Psychiatry 1995; 152:973–981Link, Google Scholar
49. Gurvits TV, Shenton ME, Hokama H, Ohta H, Lasko NB, Gilbertson MW, Orr SP, Kikinis R, Jolesz FA, McCarley RW, Pitman RK: Magnetic resonance imaging study of hippocampal volume in chronic, combat-related posttraumatic stress disorder. Biol Psychiatry 1996; 40:1091–1099Google Scholar
50. Bremner JD, Randall P, Vermetten E, Staib L, Bronen RA, Mazure C, Capelli S, McCarthy G, Innis RB, Charney DS: Magnetic resonance imaging-based measurement of hippocampal volume in posttraumatic stress disorder related to childhood physical and sexual abuse—a preliminary report. Biol Psychiatry 1997; 41:23–32Crossref, Medline, Google Scholar
51. Stein MB, Koverola C, Hanna C, Torchia MG, McClarty B: Hippocampal volume in women victimized by childhood sexual abuse. Psychol Med 1997; 27:1–9Crossref, Google Scholar
52. Dembo R, Williams L, Wothke W, Schmeidler J, Brown CH: The role of family factors, physical abuse and sexual victimization experiences in high-risk youths’ alcohol and other drug use and delinquency: a longitudinal model. Violence Vict 1992; 7:245–266Crossref, Medline, Google Scholar
53. Deykin EY, Buka SL: Prevalence and risk factors for posttraumatic stress disorder among chemically dependent adolescents. Am J Psychiatry 1997; 154:752–757Link, Google Scholar
54. Clark DB, Lesnick L, Hegedus A: Trauma and other stressors in adolescent alcohol dependence and abuse. J Am Acad Child Adolesc Psychiatry 1997; 36:1744–1751Google Scholar
55. Rivier C: Alcohol stimulates ACTH secretion in the rat: mechanisms of action and interactions with other stimuli. Alcohol Clin Exp Res 1996; 20:240–254Crossref, Medline, Google Scholar
56. Groote Veldman R, Meinders AE: On the mechanism of alcohol-induced pseudo-Cushing’s syndrome. Endocr Rev 1996; 17:262–268Crossref, Medline, Google Scholar
57. Beresford T, Arciniegas D, Rojas D, Sheeder J, Teale P, Aasal R, Sandberg E, Reite M: Hippocampal to pituitary volume ratio: a specific measure of reciprocal neuroendocrine alterations in alcohol dependence. J Stud Alcohol 1999; 60:586–588Crossref, Medline, Google Scholar
58. Castellanos FX, Giedd JN, Marsh WL, Hamburger SD, Vaituzis AC, Dickstein DP, Sarfatti SE, Vauss YC, Snell JW, Lange N, Kaysen D, Krain AL, Ritchie GF, Rajapakse JC, Rapoport JL: Quantitative brain magnetic resonance imaging in attention-deficit hyperactivity disorder. Arch Gen Psychiatry 1996; 53:607–616Crossref, Medline, Google Scholar
59. De Bellis MD, Casey BJ, Dahl RE, Birmaher B, Williamson DE, Thomas KM, Axelson DA, Frustaci K, Boring AB, Hall J, Ryan ND: A pilot study of amygdala volumes in pediatric generalized anxiety disorder. Biol Psychiatry (in press)Google Scholar
60. Ameri A: The effects of cannabinoids on the brain. Prog Neurobiol 1999; 58:315–348Crossref, Medline, Google Scholar
61. Jacobsen LK, Giedd JN, Castellanos FX, Vaituzis AC, Hamburger SD, Kumra S, Lenane MC, Rapoport JL: Progressive reduction of temporal lobe structures in childhood-onset schizophrenia. Am J Psychiatry 1998; 155:678–685Link, Google Scholar
62. Lawson JA, Nguyen W, Bleasel AF, Pereira JK, Vogrin S, Cook MJ, Bye AM: ILAE-defined epilepsy syndromes in children: correlation with quantitative MRI. Epilepsia 1998; 39:1345–1349Google Scholar
63. VanLandingham KE, Heinz ER, Cavazos JE, Lewis DV: Magnetic resonance imaging evidence of hippocampal injury after prolonged focal febrile convulsions. Ann Neurol 1998; 43:413–426Crossref, Medline, Google Scholar
64. Lezak M: Neuropsychological Assessment, 3rd ed. New York, Oxford University Press, 1995Google Scholar
65. Welsh MC, Pennington BF: Assessing frontal lobe functioning in children: views from developmental psychology. Dev Neuropsychol 1988; 4:199–230Crossref, Google Scholar
66. Ron MA, Acker RW, Shaw GW, Lishman WA: Computerized tomography of the brain in chronic alcoholism: a survey and follow-up study. Brain 1982; 105:497–514Crossref, Medline, Google Scholar
67. Pfefferbaum A, Sullivan EV, Mathalon DH, Lim KO: Frontal lobe volume loss observed with magnetic resonance imaging in older chronic alcoholics. Alcohol Clin Exp Res 1997; 21:521–529Crossref, Medline, Google Scholar
68. Shear PK, Jernigan TL, Butters N: Volumetric magnetic resonance imaging quantification of longitudinal brain changes in abstinent alcoholics. Alcohol Clin Exp Res 1994; 19:110–122Google Scholar