Reduced Concentrations of Thalamic N-Acetylaspartate in Male Patients With Schizophrenia
Abstract
OBJECTIVE: The authors measured N-acetylaspartate (a putative neuronal marker) in the right and left thalamus of 17 male patients with schizophrenia using in vivo proton magnetic resonance spectroscopic imaging (1H MRSI). METHOD: 1H MRSI was performed on 17 medicated male patients with schizophrenia and 10 male comparison subjects. Concentrations of N-acetylaspartate, creatine, and choline were determined in the thalamic regions bilaterally. RESULTS: The patients with schizophrenia demonstrated significantly lower concentrations of N-acetylaspartate than the comparison subjects in both the right and left thalamic regions. Right thalamic N-acetylaspartate and left thalamic N-acetylaspartate were significantly correlated in the patients but not in the comparison subjects. There was no association between N-acetylaspartate and duration of illness or medication dose. No group differences or lateralized asymmetries in choline or creatine were noted. CONCLUSIONS: The finding of reduced concentrations of N-acetylaspartate bilaterally suggests neuronal dysfunction and/or loss in both the right and left thalamic regions in male patients with schizophrenia.
Accumulating evidence over the years has implicated the thalamus as a brain region that may have abnormalities in structure and function in schizophrenia. This evidence includes 1) thalamic synaptic degeneration and reductions in mediodorsal thalamic neurons, mediodorsal nucleus volumes, and anterior thalamic projection neurons (1–4), 2) smaller thalamic volumes as measured by magnetic resonance imaging (MRI) (5, 6), and 3) reduced thalamic metabolic activity as measured by positron emission tomography (7, 8).
N-Acetylaspartate is the second most abundant amino acid in the central nervous system and is reliably measured by proton magnetic resonance spectroscopic imaging (1H MRSI). Reductions in N-acetylaspartate are thought to represent loss of neurons and/or axons, as well as neuronal or axonal dysfunction or damage (9–11). There have been relatively few studies using 1H MRSI specifically to investigate thalamic neuronal loss or dysfunction in schizophrenia. These preliminary investigations have reported discrepant findings (12–14), which may be caused in part by differences in the spectroscopy techniques used to examine the thalamus. Given the evidence for thalamic neuronal and synaptic loss (1–4), volumetric reduction (5, 6), and hypometabolism (7, 8), we conducted a pilot study using 1H MRSI to test the hypothesis that concentrations of thalamic N-acetylaspartate would be lower in patients with schizophrenia than in healthy comparison subjects.
METHOD
Seventeen male patients who met DSM-IV criteria for schizophrenia (mean age=35.7 years, SD=9.3) and 10 male comparison subjects (mean age=39.5 years, SD=12.8) gave written informed consent for participation in the study after the procedures had been fully explained. The procedures were approved by the University of California, San Francisco, Committee on Human Research. The diagnosis of schizophrenia was confirmed by using the Structured Clinical Interview for DSM-IV Axis I Disorders, Patient Edition (15). Comparison subjects were also assessed with the Structured Clinical Interview for DSM-IV Axis I Disorders—Non-Patient Edition (16) to rule out any major axis I diagnoses.
All subjects were right-handed. The patients with schizophrenia were all receiving stabilized doses of neuroleptic medication. The mean number of years of illness was 14.7 (SD=10.6). None of the patients with schizophrenia had a history of head injury, organic mental disorder, neurological disorder, cerebrovascular disease, major mood disorder, or anxiety disorder. None of the patients had clinically significant alcohol or substance abuse in the 12 months before the study. None of the comparison subjects had any history of substantial medical illness, head injury, neurological disorder, psychiatric disorder, or clinically significant alcohol or substance abuse. There were no significant group differences between patients and comparison subjects in age or education.
All MRSI studies were performed at the San Francisco Department of Veterans Affairs Medical Center on a Siemens 1.5-T Magnetom VISION MRI/MRS system. A standard circularly polarized head coil was used. Each subject was numerically coded for blind measurement and data processing. MRI sequences included T1-weighted scout views along with coronal magnetization-prepared rapid gradient echo and axial MRI double spin-echo images. The MRI scans from both comparison subjects and patients were evaluated by a neuroradiologist to determine if any abnormalities were present. Multislice 1H MRSI was performed according to a three-slice method (17) with the following acquisition parameters: TR/TE=1960/135 msec; 36 phase-encoding steps in each direction with circular k space sampling; 280×280-mm2 field of vision providing 8×8-mm2 in-plane resolution. MRSI data volumes were reconstructed, and the effective voxel size was 1.5 ml.
By using spectroscopic imaging display software (18), a reference image of the total 1H signal was generated together with higher resolution, spatially coregistered MRI axial double spin-echo images. A single operator blind to subject diagnosis adjusted the coregistered MRI axial image intensity and contrast to optimize visualization of the thalamus and then selected one voxel in the right and one voxel in the left mediodorsal region of the thalamus for each subject. Each voxel was located entirely within the thalamus to minimize any metabolite contamination from adjacent CSF or gray and white matter outside of the thalamus.
The voxel selections for each subject were verified by a neuroradiologist as being primarily in the mediodorsal region of the thalamus on the spatially MRI coregistered image. This voxel selection method was performed on two separate occasions by a single operator on 10 subjects studied twice to obtain intraoperator reliabilities of 0.99 for both the right and left mediodorsal thalamic voxels, with test-retest reliabilities of 0.93 and 0.94 for the right and left mediodorsal thalamic voxels, respectively. 1H spectra from these voxels were fit by an automated curve-fitting software program developed in our laboratory (19). Absolute integrals were corrected for differential head coil loading by using the transmitter reference amplitude for each subject to obtain concentration estimates of N-acetylaspartate, choline, and creatine in institutional units.
Repeated measures analysis of variance (ANOVA) was used for data analysis. The dependent variable was the concentration estimate for each metabolite (N-acetylaspartate, choline, or creatine); group was the between-subjects factor; and side (left versus right) was the within-subjects repeated measures factor. Regression analysis was used to examine the association between N-acetylaspartate and duration of illness and neuroleptic dose. To correct for multiple comparisons, the criterion of significance for ANOVA was set at p<0.02.
RESULTS
No abnormalities were noted on the MRI images of the patients or the healthy comparison subjects. The patients with schizophrenia demonstrated significantly lower concentrations of N-acetylaspartate than the comparison subjects in both the right thalamus (mean=4.44, SD=0.83, in patients with schizophrenia versus mean=5.29, SD=0.66, in comparison subjects) and the left thalamus (mean=4.71, SD=0.71, in patients with schizophrenia versus mean=5.44, SD=0.77, in comparison subjects) (F=8.7, df=1, 25, p=0.007).
FIGURE 1. presents a scatterplot of the individual N-acetylaspartate values for each subject. Because N-acetylaspartate ratios are often reported in MRS studies, N-acetylaspartate/creatine was also examined and found to be lower in the patients with schizophrenia than the comparison subjects in both the right thalamus (mean=2.20, SD=0.27, in patients with schizophrenia versus mean=2.86, SD=0.97, in comparison subjects) and the left thalamus (mean=2.34, SD=0.36, in patients with schizophrenia versus mean=2.85, SD=1.26, in comparison subjects) (F=4.6, df=1, 25, p=0.04).
Choline levels were not significantly different between groups in the right thalamus (mean=0.72, SD=0.19, in patients with schizophrenia versus mean=0.83, SD=0.14, in comparison subjects) or the left thalamus (mean=0.72, SD=0.19, in patients with schizophrenia versus mean=0.76, SD=0.13, in comparison subjects) (F=1.3, df=1, 25, p=0.27).
Creatine was also not significantly different between groups in the right thalamus (mean=2.03, SD=0.40, in patients with schizophrenia versus mean=1.98, SD=0.47, in comparison subjects) or the left thalamus (mean=2.04, SD=0.33, in patients with schizophrenia versus mean=2.07, SD=0.51, in comparison subjects) (F=0.004, df=1, 25, p=0.95).
There was no lateralized asymmetry for either N-acetylaspartate, choline, or creatine in either the patients with schizophrenia or the comparison subjects. There was a significant positive correlation between left and right N-acetylaspartate concentrations in the patients with schizophrenia (F=15.11, df=1, 15, r=0.71, p=0.002) but not in the comparison subjects (F=1.61, df=1, 8, r=0.41, p=0.24). There were no significant correlations between N-acetylaspartate and either duration of illness or neuroleptic dose in chlorpromazine equivalents.
DISCUSSION
The primary findings of this study are significantly lower concentrations of N-acetylaspartate in both the right and left thalamus in medicated male patients with schizophrenia than in healthy comparison subjects. These results suggest bilateral thalamic neuronal/axonal dysfunction or loss in schizophrenia and are consistent with previous neuropathological and neuroimaging studies demonstrating reductions in thalamic neurons (1, 3), thalamic volumes (5, 6), and thalamic metabolic activity (7, 8). The N-acetylaspartate findings are also consistent with a previous single-voxel 1H MRS study that reported a strong trend toward lower N-acetylaspartate concentrations in the left thalamus (12) and a postmortem MRS study that reported a tendency toward lower N-acetylaspartate concentrations in the left thalamus (20).
Our N-acetylaspartate findings are particularly noteworthy given that the mediodorsal and anterior thalamic nuclei have important connections to the prefrontal cortex, cingulate cortex, and hippocampus, all of which have been implicated in the pathophysiology of schizophrenia (21).
The discrepancy between the findings of the present study and those of previous proton MRSI studies that did not demonstrate any decrease in thalamic N-acetylaspartate (13, 14) may be the results of differences in voxel selection. The present study obtained N-acetylaspartate measures from a single voxel in each of the right and left thalami, whereas the previous proton MRSI studies (13, 14) averaged N-acetylaspartate measures from multiple voxels containing thalamic tissue to obtain a single N-acetylaspartate measure for each of the right and left thalami. A similar discrepancy in N-acetylaspartate findings has been noted in the anterior cingulate region: selection of a single voxel on each side yielded lower concentrations of N-acetylaspartate in patients with schizophrenia than in healthy comparison subjects (22), whereas selection of numerous voxels to obtain averaged N-acetylaspartate measures for each side did not yield any group differences (13, 14). It is conceivable that obtaining an averaged N-acetylaspartate measure from numerous thalamic voxels may fail to detect a more localized abnormality in neuronal integrity and, consequently, N-acetylaspartate, within the thalamus. In support of such a notion for the thalamus are several MRI studies of thalamic area or volumes that have noted significantly lower values for specific thalamic regions but not in total thalamic area or volume for patients with schizophrenia than for control subjects (5, 7, 8, 23).
The second finding of this study is the significant positive correlation between the right and left thalamic N-acetylaspartate measures in the group with schizophrenia but not in the comparison group. This suggests a strong association between neuronal dysfunction or loss in the right and left thalamic regions. One interpretation of this finding is that whatever neuropathological or neurodevelopmental process results in thalamic neuronal dysfunction or loss appears to affect both right and left sides to the same extent in a given individual with schizophrenia.
Although the effects of neuroleptic medication on thalamic N-acetylaspartate have not been thoroughly investigated, 1H MRSI studies of both medicated (14) and unmedicated (13) patients with schizophrenia revealed no significant differences in thalamic N-acetylaspartate measures between medicated and unmedicated subjects. Another postmortem study (24) failed to find significant differences in regional measures of N-acetylaspartate in normal control subjects compared with neuroleptic-treated control subjects, which also argues against a significant neuroleptic effect on regional N-acetylaspartate measures in the brain. Thus, it appears unlikely that neuroleptic medication was responsible for the lower concentrations of N-acetylaspartate.
In addition, spectroscopic voxels typically contain varying mixtures of gray matter, white matter, and CSF (partial volume effect), which often complicates the interpretation of any observed changes in the metabolite concentrations. The selected voxels in this study were situated entirely within the thalamus, thus minimizing any CSF contribution to the concentration estimates. Furthermore, current MRI tissue segmentation techniques used to derive estimates of N-acetylaspartate from pure gray matter, white matter, and CSF compartments within each MRSI voxel (25) are unable reliably to differentiate between more subtle gray and white matter compartments within structures such as the thalamus. Therefore, the N-acetylaspartate concentration estimates reflect N-acetylaspartate from both the gray and white matter of the thalamus.
Received Feb. 24, 1999; revision received Sept. 12, 1999; accepted Sept. 28, 1999. From the Magnetic Resonance Unit and Psychiatry Service, VA Medical Center, San Francisco; and the Departments of Radiology and Psychiatry, University of California, San Francisco. Address reprint requests to Dr. Deicken, Psychiatry Service, 116-N, VA Medical Center, 4150 Clement St., San Francisco, CA 94121; [email protected] (e-mail). Supported by a 1997 Stanley Foundation Research Award from the National Alliance for the Mentally Ill and NIMH grant MH-58244 (Dr. Deicken).
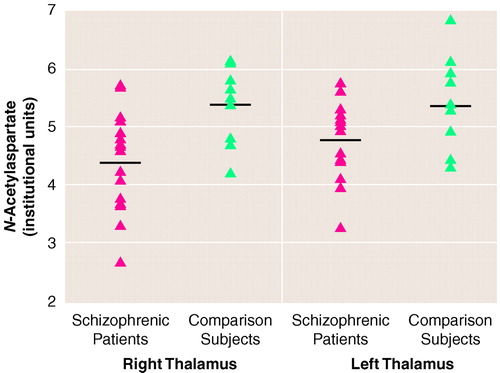
FIGURE 1. Thalamus N-Acetylaspartate Concentrations in 17 Patients With Schizophrenia and 10 Healthy Comparison Subjectsa
aHorizontal lines indicate mean N-acetylaspartate values. Data points may overlap. Two subjects in each group had identical values.
1. Pakkenberg B: Pronounced reduction of total neuron number in mediodorsal thalamic nucleus and nucleus accumbens in schizophrenics. Arch Gen Psychiatry 1990; 47:1023–1028Google Scholar
2. Pakkenberg B: The volume of the mediodorsal thalamic nucleus in treated and untreated schizophrenia. Schizophr Res 1992; 7:95–100Crossref, Medline, Google Scholar
3. Danos P, Baumann B, Bernstein HG, Franz M, Stauch R, Northoff G, Krell D, Falkai P, Bogerts B: Schizophrenia and anteroventral thalamic nucleus: selective decrease of parvalbumin-immunoreactive thalamocortical projection neurons. Psychiatry Res 1998; 82:1–10Crossref, Medline, Google Scholar
4. Blennow K, Davidsson P, Gottfries CG, Ekman R, Heilig M: Synaptic degeneration in thalamus in schizophrenia. Lancet 1996; 348:692–693Crossref, Medline, Google Scholar
5. Andreasen NC, Arndt S, Swayze V II, Cizadlo T, Flaum M, O’Leary D, Ehrhardt JC, Yuh WTC: Thalamic abnormalities in schizophrenia visualized through magnetic resonance image averaging. Science 1994; 266:294–298Crossref, Medline, Google Scholar
6. Staal WG, Hulshoff Pol HE, Schnack H, van der Schot AC, Kahn RS: Partial volume decrease of the thalamus in relatives of patients with schizophrenia. Am J Psychiatry 1998; 155:1784–1786Google Scholar
7. Buchsbaum MS, Someya T, Teng CY, Abel L, Chin S, Najafi A, Haier R, Wu J, Bunney WE Jr: PET and MRI of the thalamus in never-medicated patients with schizophrenia. Am J Psychiatry 1996; 153:191–199Link, Google Scholar
8. Hazlett EA, Buchsbaum MS, Byne W, Wei TC, Spiegel-Cohen J, Geneve C, Kinderlehrer R, Haznedar MM, Shihabuddin L, Siever LJ: Three-dimensional analysis with MRI and PET of the size, shape, and function of the thalamus in the schizophrenic spectrum. Am J Psychiatry 1999; 156:1190–1199Google Scholar
9. Tsai G, Coyle JT: N-Acetylaspartate in neuropsychiatric disorders. Prog Neurobiol 1995; 46:531–540Crossref, Medline, Google Scholar
10. De Stefano N, Matthews PM, Arnold DL: Reversible decreases in N-acetylaspartate after acute brain injury. Magn Reson Med 1995; 34:721–727Crossref, Medline, Google Scholar
11. Hugg JW, Kuzniecky RI, Gilliam FG, Morawetz RB, Fraught RE, Hetherington HP: Normalization of contralateral metabolic function following temporal lobectomy demonstrated by 1H magnetic resonance spectroscopic imaging. Ann Neurol 1996; 40:236–239Crossref, Medline, Google Scholar
12. Heimberg C, Komoroski RA, Lawson WB, Cardwell D, Karson CN: Regional proton magnetic resonance spectroscopy in schizophrenia and exploration of drug effect. Psychiatry Res 1998; 83:105–115Crossref, Medline, Google Scholar
13. Bertolino A, Callicott JH, Elman I, Mattay VS, Tedeschi G, Frank JA, Breier A, Weinberger DR: Regionally specific neuronal pathology in untreated patients with schizophrenia: a proton magnetic resonance spectroscopic imaging study. Biol Psychiatry 1998; 43:641–648Crossref, Medline, Google Scholar
14. Bertolino A, Nawroz S, Mattay VS, Barnett AS, Duyn JH, Moonen CT, Frank JA, Tedeschi G, Weinberger DR: Regionally specific pattern of neurochemical pathology in schizophrenia as assessed by multislice proton magnetic resonance spectroscopic imaging. Am J Psychiatry 1996; 153:1554–1563Google Scholar
15. First MB, Spitzer RL, Gibbon M, Williams JBW: Structured Clinical Interview for DSM-IV Axis I Disorders, Patient Edition (SCID-P), version 2. New York, New York State Psychiatric Institute, Biometrics Research, 1995Google Scholar
16. First MB, Spitzer RL, Gibbon M, Williams JB: Structured Clinical Interview for DSM-IV Axis I Disorders—Non-Patient Edition (SCID-I/NP), version 2.0. New York, New York State Psychiatric Institute, Biometrics Research, 1996Google Scholar
17. Rooney WD, Miller RG, Gelinas D, Schuff N, Maudsley AA, Weiner MW: Decreased N-acetylaspartate in motor cortex and corticospinal tract in ALS. Neurology 1998; 50:1800–1805Google Scholar
18. Maudsley AA, Lin E, Weiner MW: Spectroscopic imaging display and analysis. Magn Reson Imaging 1992; 10:471–485Crossref, Medline, Google Scholar
19. Soher BJ, Young K, Govindaraju V, Maudsley AA: Automated spectral analysis, III: application to in vivo proton MR spectroscopy and spectroscopic imaging. Magn Reson Med 1998; 40:822–831Crossref, Medline, Google Scholar
20. Omori M, Pearce J, Komoroski RA, Griffin WST, Mrak RE, Husain MM, Karson CN: In vitro 1H-magnetic resonance spectroscopy of postmortem brains with schizophrenia. Biol Psychiatry 1997; 42:359–366Crossref, Medline, Google Scholar
21. Jones EG: Cortical development and thalamic pathology in schizophrenia. Schizophr Bull 1997; 23:483–501Crossref, Medline, Google Scholar
22. Deicken RF, Zhou L, Schuff N, Weiner MW: Proton magnetic resonance spectroscopy of the anterior cingulate region in schizophrenia. Schizophr Res 1997; 27:65–71Crossref, Medline, Google Scholar
23. Jernigan TL, Zisook S, Heaton RK, Moranville JT, Hesselink JR, Braff DL: Magnetic resonance imaging abnormalities in lenticular nuclei and cerebral cortex in schizophrenia. Arch Gen Psychiatry 1991; 48:881–890Crossref, Medline, Google Scholar
24. Tsai G, Passani LA, Slusher BS, Carter R, Baer L, Kleinman JE, Coyle JT: Abnormal excitatory neurotransmitter metabolism in schizophrenic brains. Arch Gen Psychiatry 1995; 52:829–836Crossref, Medline, Google Scholar
25. MacKay S, Ezekiel F, Di Sclafani V, Meyerhoff DJ, Gerson J, Norman D, Fein G, Weiner MW: Alzheimer disease and subcortical ischemic vascular dementia: evaluation by combining MR imaging segmentation and H-1 MR spectroscopic imaging. Radiology 1996; 198:537–545Crossref, Medline, Google Scholar