Adverse Subjective Experience With Antipsychotics and Its Relationship to Striatal and Extrastriatal D 2 Receptors: a PET Study in Schizophrenia
Abstract
OBJECTIVES: Antipsychotic medications improve psychosis but often induce a state of dysphoria in patients. Blockade of the dopamine D 2 receptors, which is thought to mediate their efficacy, has also been implicated in producing this adverse subjective experience. The authors present the first double-blind controlled study to examine the relationship between striatal and extrastriatal dopamine D 2 receptor binding potential and occupancy values and adverse subjective experience. Method: Patients with recent-onset psychosis (N=12) were randomly assigned to low or high doses of olanzapine or risperidone. Subjective experiences, motor side effects, and striatal and extrastriatal dopamine D 2 receptors (determined with ([ 11 C]raclopride and [ 11 C]FLB 457 PET scans, respectively) were evaluated after 2 weeks of continuous antipsychotic treatment. Results: Higher dopamine D 2 receptor occupancy and binding potentials in the striatal (dorsal and ventral), temporal, and insular regions were associated with subjective experience. The finding was confirmed with two convergent methods of analysis (region-of-interest and voxel-based statistics), and the same relationship was observed using two different dopamine receptor measures (observed binding potential values and age- and sex-corrected occupancy values). Conclusions: Higher D 2 receptor occupancy is associated with negative subjective experience in patients taking risperidone or olanzapine. These negative subjective effects may be related to the high discontinuation rates seen in usual practice. Understanding the neurobiological mechanism of these negative subjective experiences and developing antipsychotics with novel (i.e., non D 2 ) mechanisms may be critical in improving the treatment of psychosis.
Antipsychotic medications have been the first-line treatment for psychotic disorders for nearly half a century (1) . Despite this long history of use, many patients dislike taking the medications and are eager to stop them (2) . As early as 1957 it was reported that 25% of normal subjects given only 50 mg of chlorpromazine experienced “inability to think, tiredness, sleepiness and generally unpleasant feelings” (3) . Over the years a number of reports have commented on this adverse subjective experience of antipsychotics using a diversity of terms: “behavioral toxicity,” “neuroleptic dysphoria,” “akinetic depression,” “subjective extrapyramidal side effects,” and “neuroleptic-induced deficit syndrome” (reviewed by Voruganti and Awad [4] ). While these adverse subjective effects are well documented and it is recognized that subjective experiences are key factors for adherence and recovery (2) , little is known about their underlying neurobiology.
In recent years, the disruption of the dopamine system has been associated with these subjective experiences (4) . For example, alpha-methyl paratyrosine (AMPT)—an agent that blocks catecholamine synthesis and decreases dopamine levels temporarily—has been shown to produce reversible dysphoria, decrease in happiness, and tiredness in healthy volunteers (5 , 6) and in patients with schizophrenia (7) . Further, it has been demonstrated that high levels of dopamine D 2 blockade (as measured using SPECT) is associated with dysphoric experiences (8 , 9) or depression (10) in patients with psychosis treated with antipsychotic medications. For example, de Haan used the Subjective Well-Being Under Neuroleptics scale, which reliably measures the subjective experience associated with the use of antipsychotics, and found a significant association between subjective experience and striatal D 2 receptor occupancy in schizophrenic patients clinically stable on regimens of atypical antipsychotics. Even in the absence of extrapyramidal symptoms, higher striatal D 2 receptor occupancy by olanzapine and risperidone was related to worse subjective experience, more severe negative symptoms, and depression.
While the aforementioned data implicate a blockade of dopamine transmission, particularly at the dopamine D 2 receptor, as a precursor of adverse subjective effects, all of these studies have examined only the “striatal” dopamine D 2 receptors. Dopamine D 2 receptors are expressed in several brain regions (thalamus, prefrontal cortex, temporal cortex) beyond the striatum (11) , and these “extrastriatal” regions are of particular relevance to subjective experience (12) . While the striatal regions have been associated with the motor side effects of antipsychotic medications, it is the thalamic, prefrontal, and limbic regions that are more directly implicated in cognition, reward, and motivation (13 – 16) . However, measuring extrastriatal D 2 receptors poses a technical challenge, since the levels of dopamine D 2 receptors are much lower (a tenth to a hundredth) than in the striatum (11) . [ 11 C]FLB 457 is a high affinity ligand that can reliably measure regions with low receptor density (i.e., extrastriatal regions), but it does not reach equilibrium in the striatal regions (i.e., high density) within the 60–90 minute time window that is feasible with [ 11 C] ligands (17 , 18) , hence necessitating [ 11 C]raclopride scans.
In light of these developments, we present the results of the first systematic, double-blind, prospectively controlled study examining the relationship between striatal and extrastriatal D 2 blockade and the adverse subjective experience induced by antipsychotics.
Method
Subjects
Eligible subjects were included after they provided written informed consent using forms and procedures approved by the University of Toronto Human Subjects Review Committee. Inclusion criteria were 1) age 16–55, 2) meeting DSM-IV criteria for schizophrenia, schizoaffective disorder, or schizophreniform disorder; 3) no history of antipsychotic treatment or treatment for less than 4 weeks for the current episode and drug free for at least 2 weeks; 4) minimum Positive and Negative Syndrome Scale (PANSS) total score of 55 with scores above 3 on at least two positive subscale items or above 4 on one item; and 5) no major medical or neurological illness or history of major head injury. Subjects were excluded if they 1) had a lifetime history or current substance dependence or abuse; 2) were refractory to antipsychotic treatment as defined by history of clozapine use or a history of poor response. The diagnoses were established using the Mini International Neuropsychiatric Interview (MINI). Patients were randomly assigned to a low-dose treatment with olanzapine (2.5 mg/day) or risperidone (1 mg/day) or standard-dose treatment with olanzapine (15 mg/day) or risperidone (4 mg/day). Subjects were scanned on average after 15 days (SD=2), and the subjective and objective clinical ratings were completed by raters blind to dose and occupancy.
Of the patients that met the aforementioned criteria, those who successfully completed [ 11 C]raclopride and [ 11 C]FLB 457 scans as well as subjective and objective clinical ratings on the day of the PET scan were included in the present study. Objective ratings were obtained using Clinical Global Impression (CGI) ratings, PANSS, Barnes Akathisia Scale, and the Simpson-Angus Rating Scale (for presence of parkinsonism). The subjective experience with the use of antipsychotics was captured with the Subjective Well-Being Under Neuroleptics scale. The Subjective Well-Being Under Neuroleptics scale has five subscales: mental functioning, self-control, emotional regulation, physical functioning, and social integration. Items are clearly formulated and easy to understand so that even the most cognitively disturbed patients are able to fill out the questionnaire within 5–10 minutes. The 20-item version has shown adequate psychometric properties (Cronbach alpha coefficient 0.92) (19) .
PET Analysis
Extrastriatal ([ 11 C]FLB 457) and striatal ([ 11 C]raclopride) dopamine D 2 receptor occupancy were assessed after 15 days (SD=2) of continuous antipsychotic treatment, on the same day, 12 and 14 hours after the last administered dose, respectively. The [ 11 C]FLB 457 PET scans for extrastriatal D 2 receptors were obtained immediately following injection of 9.75 mCi (SD=0.65) of high specific-activity [ 11 C]FLB 457 via single bolus intravenous injection, with emission scans collected every minute for the first 15 minutes and then every 5 minutes until the end of the study at 90 minutes. [ 11 C]Raclopride was injected using a bolus plus infusion technique (20) . Fifteen mCi were diluted in 20 ml of saline and 11.8 ml were injected as a slow bolus followed by a constant infusion at the rate of 0.11 ml/min until the end of the scan acquisition. Following a brief transmission scan for attenuation correction of the emission scans, a continuous series of emission scans were obtained every minute for the first 15 minutes and then every 5 minutes until the end of the study at 75 minutes. PET scanning was conducted using a brain-only PET scanner (GEMS PC2048-Plus), which provides 15 slices of 6.5-mm thickness with an in-plane resolution of about 5 to 6 mm in air. Patients were scanned lying down and with fixation of the head achieved using a thermoplastic facemask (Tru-Scan Imaging, Annapolis, Md.), allowing for accurate repositioning across scans.
Regions of Interest Based Analysis
To permit accurate delineation of the brain regions for data analysis, each patient had an MRI scan done using a GE-Signa 1.5-Tesla scanner. A fast spin echo axial T1-weighted sequence with 1.5-mm thickness and proton density image with 3-mm slice thickness were performed. The MRI scan of each patient was coregistered to his or her PET scan using RView8 software (with one exception when regions of interest were delineated on the sum PET image because one subject did not undergo an MRI scan). The regions of interest used in the analysis were the striatum (caudate/putamen) and extrastriatal regions (frontal cortex, temporal cortex, and thalamus). The cerebellum was used as a reference region in the analysis of both striatal and extrastriatal receptor occupancy studies. The regions of interest were delineated using Alice 3.0 (Perceptive Systems), which allows the rater to draw regions of interest on an MRI coregistered with the summed PET images (representing averaged images of the dynamic time series) and to manually correct for head movement. The regions of interest were drawn by a single rater (blind to treatment allocation) using previously described boundaries on two axial slices for the cerebellum, two axial slices for the striatum, two axial slices for the frontal and temporal cortices, and one axial slice for the thalamus. The regions of interest were drawn such that their volume was larger than twice the full width at half maximum to minimize errors due to partial volume effects (21) .
Striatal D 2 receptor binding potential and extrastriatal D 2 receptor binding potential were calculated from the time activity curves generated from the [ 11 C]raclopride and [ 11 C]FLB 457 scans using the simplified reference tissue model, using the cerebellum as the reference tissue and the striatal, frontal cortex, temporal cortex, and thalamus as the regions of interest (17 , 22) . Receptor occupancy was then calculated as the percentage reduction of receptor binding potential with drug treatment relative to baseline: 100 x (1 – [BP drug scan /BP EstBaseline ]). Because of the nature of the illness and the urgency to start treatment, we were unable to obtain drug-free baseline scans in this study. Therefore, the baseline was estimated by using age-corrected measures of binding potential from a previously collected dataset of 17 [ 11 C]raclopride scans and 13 [ 11 C]FLB 457 scans of normal subjects. The same rater using the same methodology to ensure within-study consistency analyzed the data from these control subjects.
Voxel-Based Analysis
The processing and analysis of binding potential maps were performed using statistical parametric mapping SPM2 software (SPM2, Wellcome Department of Cognitive Neurology, London). Briefly, the T1 image of each patient was coregistered to its corresponding original PET. The coregistered T1 images were spatially (stereotactically) normalized using the nearest neighbor algorithm into the T1 template from the Montreal Neurological Institute (MNI) then applying the normalization parameter to the binding potential images. These images in the MNI space were masked for the a priori regions (whole striatum for [ 11 C]raclopride scans and frontal, temporal, parietal, and insular regions for [ 11 C]FLB 457 scans). The design matrix was a simple regression analysis, where the binding potential voxelwise for every image were the dependent variables, and the independent variables were the Subjective Well-Being Under Neuroleptics scale scores. These correlations generated t statistic parametric maps. The statistical threshold for a priori regions was an uncorrected p value of <0.01 and a corrected p value of <0.05. The corrected p value was obtained using the false discovery rate approach to control error rates and avoid random findings. This correction was performed in a small volume of 10 mm from the maximal voxel of each cluster. This stringent criterion was chosen to minimize errors due to partial volume effects or spurious SPM findings. Results were graphically presented in the Talairach-Tournoux coordinate system and overlaid on the MNI template, T1 MRI.
Results
Twelve patients (11 men and one woman) were included in the present study, of whom eight were neuroleptic naive before randomization. Their mean age was 27 years (range=17–45), and they had a mean duration of psychotic symptoms of 98 weeks (range=4–530). Nine of these patients met the criteria for schizophrenia and three met the criteria for provisional schizophreniform disorder at the time of inclusion in the study. Five subjects received risperidone (either 1 mg [N=2] or 4 mg [N=3]) and seven received olanzapine (either 2.5 mg [N=3] or 15 mg [N=4]). On the day of the PET scan the patients exhibited mild/moderate severity according to the CGI severity ratings (mean score=3.7, SD=0.67), and the mean PANSS score was 65.90 (SD=8.3). The mean PANSS positive and negative symptom scores were 15.10 (SD=2.6) and 15.60 (SD=5.3), respectively. Simpson-Angus Rating Scale score was 3.62 (SD=4.86), and the Barnes Akathisia Scale score was 0.37 (SD=1.09). The Subjective Well-Being Under Neuroleptics scale total score was 87.25 (SD=17.51) and was not associated with PANSS positive symptom scores (r= 0.11, p=0.78). Subjective Well-Being Under Neuroleptics total score was significantly correlated with Simpson-Angus Rating Scale score (r=–0.71, p=0.047) but not with the Barnes Akathisia Scale score (r= –0.34, p=0.37). Two subjects who had the highest Simpson-Angus Rating Scale scores received benztropine.
The measured D 2 occupancies ranged from 50%–91% in striatal and 4%–95% in the different extrastriatal regions. The Subjective Well-Being Under Neuroleptics scale total score was significantly associated with striatal occupancy (r=–0.66, p=0.01 [ Figure 1 ]) and temporal occupancy (r=–0.76, p=0.003 [ Figure 2 ]) but not frontal or thalamic occupancy. These associations remained unchanged when subjects were divided by those that were treated with either risperidone or olanzapine, excluded those that received previous antipsychotic treatment, or those with the highest Simpson-Angus Rating Scale scores. To further investigate these associations between striatal and temporal occupancy with subjective experience, we performed stepwise regression analysis with the Subjective Well-Being Under Neuroleptics subscales to avoid multiple comparisons. A significant association (F=12.75, df=1, 10, p=0.005) was seen between striatal blockade and mental functioning (β=-0.74, t=–3.57, p=0.005) but not self-control (β=–0.05, t=–0.20, p=0.84), emotional regulation (β=–0.11, t=–0.36, p=0.72), physical functioning (β=–0.10, t=–0.22, p=0.82), or social integration (β= –0.15, t=–0.50, p=0.62). A significant association (F=8.73, df=1, 10, p=0.01) was seen between temporal blockade and emotional regulation (β=–0.68, t=–2.95, p=0.01), but self-control (β=–0.01, t=–0.03, p=0.97), mental functioning (β=–0.29, t=–0.83, p=0.42), physical functioning (β= –0.14, t=–0.43, p=0.67), and social integration (β=–0.30, t=–1.03, p=0.32) were excluded from the model.

a The data points “A,” “B,” and “C” represent the subjects profiled in the Patient Perspectives.

a The data points “A,” “B,” and “C” represent the subjects profiled in the Patient Perspectives.
Since occupancy is a derived measure, i.e., it is obtained after correcting individual measured binding potential for estimated baseline levels, we wanted to examine if the raw binding potential data also correlated with Subjective Well-Being Under Neuroleptics scale score. The data showed that total score was significantly associated with striatal binding potential (r=0.63, p<0.03) and temporal binding potential (r=0.63, p=0.02) but not associated with frontal (r=0.34, p=0.30) or thalamic binding potentials (r=0.32, p=0.30). To obtain a finer-grained analysis of the subregions within the striatal and extrastriatal regions that correlated with Subjective Well-Being Under Neuroleptics score, we examined the correlation between voxel-based binding potential maps and score. Within the striatal region, we found that the total score on the Subjective Well-Being Under Neuroleptics scale was positively and significantly correlated with both the dorsal (right and left) regions (r=0.78, p=0.004 and r=0.78, p=0.004, respectively) and the ventral regions (r=0.77, p=0.005) of the striatum. Within the extrastriatal region, we found a strong correlation between insular cortex binding potential and score (r=0.75, p=0.007). The higher the binding potential in the insular cortex (i.e., lower occupancy by the antipsychotic drug), the higher the well-being. Smaller regions in the right dorsolateral prefrontal cortex (r=0.80, p=0.03) and the left temporal pole (r=0.77 p=0.005) also showed significant relationships ( Table 1 and Figure 3 ).

a Bars on the right represent t scores. The data points “A,” “B,” and “C” represent the subjects profiled in the Patient Perspectives.
Discussion
The study provides the first insight into the relationship between the patients’ subjective experience with antipsychotics and blockade of the dopamine D 2 receptors in different brain regions. Higher dopamine D 2 receptor occupancy in the striatal (dorsal and ventral), temporal, and insular regions was negatively associated with subjective experience when patients were receiving stable doses of antipsychotic medication. The robustness of this finding is supported by the fact that the same association is observed with both drugs under study (risperidone and olanzapine) using two methods of analysis (region-of-interest and voxel-based statistics) and two different dopamine receptor measures (observed binding potential values and age- and sex-corrected occupancy values).
The limitations of the present study are discussed to provide a context for further discussion. First, the sample size in the study was small. However, previous data that investigated the correlations between either AMPT or antipsychotic-induced dysphoria ranged from r=0.53 (23) to r=0.82 (7) . Using the standard 80% power and the conventional reliability to reject the null hypothesis (i.e., p<0.05), our study’s size was adequate to detect a significant correlation between those ranges. Finally, despite the small size we found converging results with several different methods, thereby enhancing the internal consistency of our findings. Limitations also exist in our estimates of D 2 receptor occupancies. To estimate binding potential for [ 11 C]FLB 457 we used the Simplified Reference Tissue Model (SRTM), a model that assumes that the cerebellum has no specific binding (22) . However, it has been shown that there may be a small, but measurable, signal arising from D 2 /D 3 receptor binding by [ 11 C]FLB 457 in the cerebellum. It is estimated that the error (a potential underestimation of occupancies) induced by a displaceable [ 11 C]FLB 457 signal in the cerebellum would range from 2%–5% for true occupancies in the range of 70%–90% (24) . In addition, it has been shown (25 – 27) that binding potential for dopamine D 2 /D 3 receptors in the thalamus is 8%–25% lower in patients with schizophrenia as compared with healthy volunteers. These differences in baseline could lead to a potential overestimation of occupancies that was estimated to range from 5% (SD=0.7) to 15% (SD=2.21). However, since such a group baseline difference by itself does not change the relative order of occupancies, it does not alter the associations with Subjective Well-Being Under Neuroleptics scale scores reported herein. While this simulation gives some reassurance, only a direct study measuring the baseline in individual patients can conclusively rule out the contributions of such a bias. In addition, the fact that we found a concordant association when the analysis involved the patient’s own measured binding potential and subjective experiences provides additional reassurance that this is not a false positive finding. Finally, we only investigated atypical antipsychotics, while typical antipsychotics have been associated with worse subjective experiences (19) .
In the present work we replicated studies by de Haan and coworkers who reported an association between striatal D 2 blockade and Subjective Well-Being Under Neuroleptics score using SPECT (8 , 9 , 23) . The present results are also consistent with the studies that have shown that disruption of the dopamine function produces dysphoria, decreases happiness, and provokes tiredness in healthy volunteers (6 , 28) and in drug-free schizophrenic patients (7) . We extend those initial findings by showing that striatal blockade of the D 2 receptors was associated with impaired mental functioning and temporal blockade with altered emotional regulation. This is not surprising since the dopamine action has been associated with cognition, motivation, emotion, and action (12) . What is also new in the present study is the fact that the ventral portion of the striatum, as well as the insular cortex (which is also part of the limbic system) (29) , were shown to have a specific association with Subjective Well-Being Under Neuroleptics score. It has traditionally been assumed that the same mechanism (D 2 blockade) that leads to the motor side effects may lead to the dysphoric subjective experiences reported by patients. The motor side effects of antipsychotics are typically associated with the dorsal region of the striatum (30) . We found that the dysphoric effects correlate with not only the dorsal regions but also the ventral striatum and the insular cortex. These latter regions are considered parts of the “limbic” circuitry and can be plausibly linked to a role in subjective wellness. The ventral portion of the striatum (which is thought to be the analogue of the nucleus accumbens in animals) is directly implicated in reward and motivation (15 , 31) and is key to many of the nonmotor actions of antipsychotics in animals (i.e., conditioned avoidance response models) (32) . It is well documented that increased dopamine release in the nucleus accumbens is associated with the rewarding effects accompanying the acute administration of most drugs of abuse (33 – 35) . For example, euphoric responses elicited by amphetamine were inversely correlated with change in ventral striatum binding potential in healthy volunteers (35) . In addition, the insular cortex, which has modest concentrations of dopamine D 2 receptors as measured in autoradiography studies in human brains (36) , has been implicated in the integration of internal states and has been associated with feelings of sadness (37) and the subjective awareness of internal feelings (38) . While the precise role of the dopamine receptors in the insula is not known, given its key role in integrating the extrapersonal stimuli and the internal milieu (29) , it is conceivable that altered transmission in this region may be experienced as an adverse subjective effect.
Taken together, the present data suggests that dopamine D 2 blockade, which is customarily related to antipsychotic efficacy and motor side effects, is also associated with the induction of dysphoria. However, there was no association between PANSS positive symptom and Subjective Well-Being Under Neuroleptics scores, suggesting that psychopathology and adverse subjective experiences are partially independent measures. In fact, less than 16% of the variance in Subjective Well-Being Under Neuroleptics can be explained by PANSS scores (19) . Thus, clinicians face the paradox of using drugs that will improve symptoms but at the same time may induce dysphoria and hence reduce its adherence. This may be an intrinsic problem of D 2 -blocking antipsychotics. It may be the case that antipsychotics with novel mechanisms of action, e.g., partial D 2 agonists or “dopamine stabilizers” (39) or drugs that avoid D 2 blockade altogether may be needed to avoid this dilemma. Of these options, only a partial agonist mechanism is currently clinically available (e.g., aripiprazole), and it would be interesting to see if this relationship is observed with aripiprazole.
In summary, a high proportion of patients taking antipsychotics report dysphoric experiences, and our study shows that this is associated with antipsychotic dopamine D 2 receptor blockade in different striatal and extrastriatal regions. Since this correlation was seen with atypical antipsychotics, and even in patients who do not show overt motor side effects, it seems that this negative effect may be an unavoidable consequence in many patients with high dopamine D 2 blockade. Given that these negative subjective effects may be related to the high discontinuation rates seen in clinical practice, future drug development may focus on antipsychotic drugs with a qualitatively different mechanism of action to avoid this effect.
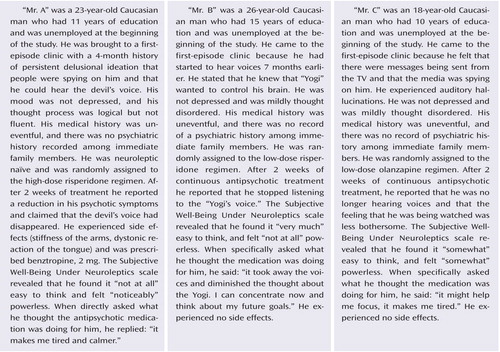
1. Kapur S, Mamo D: Half a century of antipsychotics and still a central role for dopamine D2 receptors. Prog Neuropsychopharmacol Biol Psychiatry 2003; 27:1081–1090Google Scholar
2. Marder SR: Subjective experiences on antipsychotic medications: synthesis and conclusions. Acta Psychiatr Scand Suppl 2005; 427:43–46Google Scholar
3. Hollister LE: Complications from the use of tranquilizing drugs. N Engl J Med 1957; 257:170–177Google Scholar
4. Voruganti L, Awad AG: Neuroleptic dysphoria: towards a new synthesis. Psychopharmacology (Berl) 2004; 171:121–132Google Scholar
5. Verhoeff NP, Kapur S, Hussey D, Lee M, Christensen B, Psych C, Papatheodorou G, Zipursky RB: A simple method to measure baseline occupancy of neostriatal dopamine D2 receptors by dopamine in vivo in healthy subjects. Neuropsychopharmacology 2001; 25:213–223Google Scholar
6. Verhoeff NP, Christensen BK, Hussey D, Lee M, Papatheodorou G, Kopala L, Rui Q, Zipursky RB, Kapur S: Effects of catecholamine depletion on D2 receptor binding, mood, and attentiveness in humans: a replication study. Pharmacol Biochem Behav 2003; 74:425–432Google Scholar
7. Voruganti L, Slomka P, Zabel P, Costa G, So A, Mattar A, Awad AG: Subjective effects of AMPT-induced dopamine depletion in schizophrenia: correlation between dysphoric responses and striatal D(2) binding ratios on SPECT imaging. Neuropsychopharmacology 2001; 25:642–650Google Scholar
8. de Haan L, Lavalaye J, van Bruggen M, van Nimwegen L, Booij J, van Amelsvoort T, Linszen D: Subjective experience and dopamine D2 receptor occupancy in patients treated with antipsychotics: clinical implications. Can J Psychiatry 2004; 49:290–296Google Scholar
9. de Haan L, van Bruggen M, Lavalaye J, Booij J, Dingemans PM, Linszen D: Subjective experience and D2 receptor occupancy in patients with recent-onset schizophrenia treated with low-dose olanzapine or haloperidol: a randomized, double-blind study. Am J Psychiatry 2003; 160:303–309Google Scholar
10. Bressan RA, Costa DC, Jones HM, Ell PJ, Pilowsky LS: Typical antipsychotic drugs – D(2) receptor occupancy and depressive symptoms in schizophrenia. Schizophr Res 2002; 56:31–36Google Scholar
11. Hall H, Farde L, Halldin C, Hurd YL, Pauli S, Sedvall G: Autoradiographic localization of extrastriatal D2-dopamine receptors in the human brain using [125I]epidepride. Synapse 1996; 23:115–123Google Scholar
12. Nieoullon A, Coquerel A: Dopamine: a key regulator to adapt action, emotion, motivation and cognition. Curr Opin Neurol 2003; 16(suppl 2):S3–S9Google Scholar
13. Bigliani V, Mulligan RS, Acton PD, Ohlsen RI, Pike VW, Ell PJ, Gacinovic S, Kerwin RW, Pilowsky LS: Striatal and temporal cortical D2/D3 receptor occupancy by olanzapine and sertindole in vivo: a [123I]epidepride single photon emission tomography (SPET) study. Psychopharmacology (Berl) 2000; 150:132–140Google Scholar
14. Xiberas X, Martinot JL, Mallet L, Artiges E, Loc HC, Maziere B, Paillere-Martinot ML: Extrastriatal and striatal D(2) dopamine receptor blockade with haloperidol or new antipsychotic drugs in patients with schizophrenia. Br J Psychiatry 2001; 179:503–508Google Scholar
15. Wise RA: Dopamine, learning and motivation. Nature Reviews Neuroscience 2004; 5:483–494Google Scholar
16. Pilowsky LS: Probing targets for antipsychotic drug action with PET and SPET receptor imaging. Nucl Med Commun 2001; 22:829–833Google Scholar
17. Olsson H, Halldin C, Swahn CG, Farde L: Quantification of [11C]FLB 457 binding to extrastriatal dopamine receptors in the human brain. J Cereb Blood Flow Metab 1999; 19:1164–1173Google Scholar
18. Olsson H, Farde L: Potentials and pitfalls using high affinity radioligands in PET and SPET determinations on regional drug induced D2 receptor occupancy: a simulation study based on experimental data. Neuroimage 2001; 14:936–945Google Scholar
19. Naber D, Moritz S, Lambert M, Pajonk FG, Holzbach R, Mass R, Andresen B: Improvement of schizophrenic patients’ subjective well-being under atypical antipsychotic drugs. Schizophr Res 2001; 50:79–88Google Scholar
20. Houle SKS, Hussey D, Jones C, Dasilva J, Wilson AA: Quantification of Brain Function Using PET. Edited by Myers RCV, Bailey D, Jones T. London, Academic Press Inc, 1996, pp 262–265Google Scholar
21. Bremner JD, Bronen RA, De Erasquin G, Vermetten E, Staib LH, Ng CK, Soufer R, Charney DS, Innis RB: Development and reliability of a method for using magnetic resonance imaging for the definition of regions of interest for positron emission tomography. Clin Positron Imaging 1998; 1:145–159Google Scholar
22. Lammertsma AA, Hume SP: Simplified reference tissue model for PET receptor studies. Neuroimage 1996; 4(3, pt 1):153–158Google Scholar
23. de Haan L, Lavalaye J, Linszen D, Dingemans PM, Booij J: Subjective experience and striatal dopamine D(2) receptor occupancy in patients with schizophrenia stabilized by olanzapine or risperidone. Am J Psychiatry 2000; 157:1019–1020Google Scholar
24. Olsson H, Halldin C, Farde L: Differentiation of extrastriatal dopamine D2 receptor density and affinity in the human brain using PET. Neuroimage 2004; 22:794–803Google Scholar
25. Kessler RM, Ansari MS, Riccardi P, Li R, Jayathilake K, Dawant B, Meltzer HY: Occupancy of striatal and extrastriatal dopamine D(2)/D(3) receptors by olanzapine and haloperidol. Neuropsychopharmacology 2005; 30:2283–2289Google Scholar
26. Buchsbaum MS, Christian BT, Lehrer DS, Narayanan TK, Shi B, Mantil J, Kemether E, Oakes TR, Mukherjee J: D2/D3 dopamine receptor binding with [F-18]fallypride in thalamus and cortex of patients with schizophrenia. Schizophr Res 2006; 85(1–3):232–244Google Scholar
27. Talvik M, Nordstrom AL, Olsson H, Halldin C, Farde L: Decreased thalamic D2/D3 receptor binding in drug-naive patients with schizophrenia: a PET study with [11C]FLB 457. Int J Neuropsychopharmacol 2003; 6:361–370Google Scholar
28. Fujita M, Verhoeff NP, Varrone A, Zoghbi SS, Baldwin RM, Jatlow PA, Anderson GM, Seibyl JP, Innis RB: Imaging extrastriatal dopamine D(2) receptor occupancy by endogenous dopamine in healthy humans. Eur J Pharmacol 2000; 387:179–188Google Scholar
29. Mesulam MM, Mufson EJ: Insula of the old world monkey, I: architectonics in the insulo-orbito-temporal component of the paralimbic brain. J Comp Neurol 1982; 212:1–22Google Scholar
30. Deutch AY, Lewis DA, Whitehead RE, Elsworth JD, Iadarola MJ, Redmond DE Jr, Roth RH: Effects of D2 dopamine receptor antagonists on Fos protein expression in the striatal complex and entorhinal cortex of the nonhuman primate. Synapse 1996; 23:182–191Google Scholar
31. Wise RA: Brain reward circuitry: insights from unsensed incentives. Neuron 2002; 36:229–240Google Scholar
32. Arnt J, Skarsfeldt T, Hyttel J: Differentiation of classical and novel antipsychotics using animal models. Int Clin Psychopharmacol 1997; 12(suppl 1):S9–S17Google Scholar
33. Brody AL, Olmstead RE, London ED, Farahi J, Meyer JH, Grossman P, Lee GS, Huang J, Hahn EL, Mandelkern MA: Smoking-induced ventral striatum dopamine release. Am J Psychiatry 2004; 161:1211–1218Google Scholar
34. Boileau I, Assaad JM, Pihl RO, Benkelfat C, Leyton M, Diksic M, Tremblay RE, Dagher A: Alcohol promotes dopamine release in the human nucleus accumbens. Synapse 2003; 49:226–231Google Scholar
35. Drevets WC, Gautier C, Price JC, Kupfer DJ, Kinahan PE, Grace AA, Price JL, Mathis CA: Amphetamine-induced dopamine release in human ventral striatum correlates with euphoria. Biol Psychiatry 2001; 49:81–96Google Scholar
36. Camus A, Javoy-Agid F, Dubois A, Scatton B: Autoradiographic localization and quantification of dopamine D2 receptors in normal human brain with [3H]N-n-propylnorapomorphine. Brain Res 1986; 375:135–149Google Scholar
37. Mayberg HS, Liotti M, Brannan SK, McGinnis S, Mahurin RK, Jerabek PA, Silva JA, Tekell JL, Martin CC, Lancaster JL, Fox PT: Reciprocal limbic-cortical function and negative mood: converging PET findings in depression and normal sadness. Am J Psychiatry 1999; 156:675–682Google Scholar
38. Craig AD: Human feelings: why are some more aware than others? Trends Cogn Sci 2004; 8:239–241Google Scholar
39. Tamminga CA, Carlsson A: Partial dopamine agonists and dopaminergic stabilizers in the treatment of psychosis. Curr Drug Targets CNS Neurol Disord 2002; 1:141–147Google Scholar