Amygdalar Volume and Emotional Memory in Alzheimer’s Disease
Abstract
OBJECTIVE: Everyday experience suggests that highly emotional events are often the most memorable. Experimental work in animals and humans has demonstrated that the amygdaloid complex plays a crucial role in emotional memory, i.e., memory of events arousing strong emotions. The aim of this study was to elucidate the relationship between medial temporal damage and impaired memory of real-life emotional events in patients with Alzheimer’s disease. METHOD: In 36 patients with probable Alzheimer’s disease who experienced the 1995 earthquake in Kobe, Japan, memories of events surrounding the earthquake were examined as an index of emotional memory with the use of a semistructured interview, and amygdalar and hippocampal volumes were quantified by magnetic resonance imaging. The effects of the atrophy of these structures on recall performance were determined by multiple regression analysis. RESULTS: Irrespective of generalized brain atrophy and cognitive impairments, emotional memory was correlated more with normalized amygdalar volume (right and left averaged) than with normalized hippocampal volume. General knowledge of the earthquake was correlated with neither amygdalar nor hippocampal volume. CONCLUSIONS: The results indicate that impairment of emotional event memory in patients with Alzheimer’s disease is related to intensity of amygdalar damage and provide evidence of the amygdala’s involvement in emotional memory in humans.
It has been demonstrated in both naturalistic and experimental studies that emotional arousal enhances declarative memory in humans. Emotional memory in humans is defined as memory of events arousing emotion, which is a type of episodic memory (1, 2). Under special circumstances, these memories are also referred to as “flashbulb” memories (3, 4). Distinctive psychological attributes of emotional memory in humans have been identified (5–8). Studies of animal models involving nondeclarative emotional memory (behaviors associated with emotional situations) have indicated that the neural system underlying emotional memory involves the amygdala and structures with which it is connected (9, 10). Several studies of patients with Urbach-Wiethe disease, in which the amygdala is selectively damaged, have also indicated a central involvement of the amygdala in emotional memory in humans (2, 11–13). However, studies in humans have been limited, and the role of the amygdala in memory function is still controversial. It is difficult to examine emotional memory in experimental psychometric settings, which virtually delegate emotional context to test materials while the quality and intensity of emotional involvement in memory of real-life episodes are hardly controlled. In addition, conditions that selectively injure the amygdala are exceedingly rare. In Alzheimer’s disease, in which memory impairment is a predominant feature, damage in the medial temporal lobe, including the amygdala and hippocampus, has been considered to account for memory impairments (14–17). Nevertheless, little attention has been paid to emotional memory deficits in Alzheimer’s disease.
The Kobe earthquake (officially, the South Hyogo earthquake), measuring 7.2 on the Richter scale, hit the greater Kobe area in Japan on Jan. 17, 1995, and caused more than 6,000 deaths and enormous structural damage. For most residents, this earthquake was the strongest that they had ever experienced, and it forcibly and simultaneously exposed them to fear of death. This event was likely to be strongly emotionally charged for all who experienced it. In our previous study (18), where memories of 51 patients with Alzheimer’s disease who experienced the earthquake were examined 2 months after the disaster, we found that memories of the earthquake were relatively well retained in comparison with an event that had less emotional involvement (their first magnetic resonance imaging [MRI] examination), suggesting that extraordinary emotional involvement in the experience enhanced learning and long-term storage of memory, although the function of emotional memory was certainly impaired in these patients. In the present study, to elucidate the possible role of the medial temporal structures in emotional memory, we studied memories of the earthquake as an index of emotionally loaded event memory in patients with Alzheimer’s disease by using a semistructured interview 6–10 weeks after the disaster and correlating the patients’ recall performance with volumes of the amygdaloid complex and hippocampal formation measured by high-resolution MRI.
METHOD
All experiments were performed in compliance with the relevant laws and institutional guidelines. After a complete description of the study to the subjects and their relatives, written informed consent was obtained.
Patients with Alzheimer’s disease were recruited from those who were consecutively seen in our outpatient dementia clinic in March 1995. All patients were examined by both neurologists and psychiatrists and underwent laboratory tests, cranial MRIs, and EEG, the results of which were considered in making the diagnosis. The inclusion criteria for the study were that patients had to 1) meet the criteria of the National Institute of Neurological and Communicative Disorders and Stroke and the Alzheimer’s Disease and Related Disorders Association for probable Alzheimer’s disease (19); 2) have lived in the greater Kobe area and experienced the earthquake at home at an intensity between level 4 on the Japanese Earthquake Intensity Scale (18) (houses are jolted strongly, unstable objects topple, liquids spill out of containers, walking becomes difficult, and many people go out of their homes) and level 7 (more than 30% of buildings collapse, the ground is considerably distorted by large cracks and fissures, and slope failures and landslides take place), corresponding to levels VI to X on the Modified Mercalli Intensity Scale; and 3) have been attended by reliable informants, such as live-in family members or caregivers. The exclusion criteria were complications of other neurological diseases or physical illness, physical injury or suffering from loss of a family member or home because of the earthquake, or our inability to obtain informed consent.
Thirty-six patients (27 women and nine men) were studied. Their mean age was 73.0 years (SD=8.9, range=54–87), their mean level of education was 8.4 years (SD=2.1, range=6–13), and their mean Mini-Mental State (20) score was 17.1 (SD=5.2, range=2–27). Dementia was very mild in seven patients, mild in 16 patients, moderate in nine patients, and severe in four patients as determined by the Clinical Dementia Rating (21).
To obtain normative volumetric data for comparison, 27 normal subjects (17 women and 10 men) were recruited from the community. They had normal cognitive function (Mini-Mental State score greater than 28), normal findings in physical and neurological examinations, no history of psychiatric or neurological disorders, and no abnormal findings on MRIs. The mean age of the normal subjects was 72.0 years (SD=4.2).
Emotional Memory Evaluation and Neuropsychological Assessment
Each patient’s memories of experiences of the Kobe earthquake were assessed by a semistructured interview (appendix 1) 6–10 weeks after the disaster (mean interval=59.5 days, SD=9.6). The procedure has been reported in detail elsewhere (18). In brief, three items on the questionnaire (section A, questions 1–3: event recall) were arranged to examine the patient’s recollection of earthquake events in a hierarchical order. If the subject responded correctly to question 1 or 2, a total of 3 or 2 points was given and the next question(s) was disregarded. A score of 1 was given if the subject recognized the earthquake correctly on question 3, and a score of 0 was assigned for an incorrect response or no response to any of the questions. The next three items (section B, questions 1–3: personal memory) measured the depth of memories of personal episodes concerning the earthquake. Patients’ responses were evaluated according to information from their informants. The next three items (section C, questions 1–3: general knowledge) assessed nonpersonal factual knowledge of the earthquake. In sections B and C, the number of correct responses to items (from 0 to 3) was scored. The total emotional memory score was the sum of scores of sections A, B, and C. Cognitively normal aged people responded immediately to question 1 in section A and answered correctly all of the items in sections B and C.
Memory was assessed with a Japanese version of the Wechsler Memory Scale—Revised (22). We considered the weighted sum of raw scores on the verbal memory subtests and the visual memory subtests and the total weighted sum score.
MRI Volumetry
The mean interval between the earthquake and the MRI study was 69.9 days (SD=29.9), and the mean interval between the memory survey and the MRI study was 10.5 days (SD=26.1). We measured volumes of the amygdaloid complex and hippocampal formation, as well as whole brain volume and total intracranial volume, on three-dimensional spoiled gradient echo images perpendicular to the anterior-posterior commissure plane, covering the whole calvarium. The images were generated on a 1.5-T MRI unit (Signa Advantage 5.x, General Electric Co.) (124 1.5-mm contiguous sections, field of view=220 mm, matrix=256×256 pixels, TR=14 msec, TE=3 msec, flip angle=20˚) (17, 23).
Volumes of the amygdala and hippocampus were measured with a personal computer (Power Macintosh 9500, Apple) and the National Institutes of Health image program, version 1.60 (in the public domain) with macro programs developed at our institute. We used a semiautomatic segmentation technique through manual tracing and density thresholding (23–25), thereby minimizing partial voluming and observer’s bias. The slice volume of each structure was obtained by automatically counting the number of pixels within the segmented regions and then multiplying this number by the voxel size ([220/256]2×1.5=1.108 mm3). To measure the volume of a structure, the structure’s outline together with the surrounding white matter and CSF were first manually traced with a mouse cursor. The gray matter of each structure was then extracted by density thresholding with the use of a range between minimum and maximum pixel values. The maximum value was the largest value for any pixel of the gray matter (represented by the caudate head). The minimum value was defined as one-half the sum of the mean pixel value of the gray matter and the mean value of the CSF (represented by the lateral ventricle) (17). Measurements were performed by one investigator (M.I.) who was blind to the clinical information. The amygdaloid complex was defined to include the amygdala proper, the gyrus semilunaris, and the gyrus ambiens, which constitutes the olfactory portion of the entorhinal cortex, and the hippocampal formation was defined to include the pes hippocampi, digitationes hippocampi, alveus hippocampi, dentate gyrus, and subiculum (figure 1). The details and accuracy data of this method are described elsewhere (17, 23, 26). The test-retest reliability, measured by an intraclass correlation coefficient (ICC) derived from three repeated measurements by one rater (E.M.), under blind conditions, in 10 randomly selected subjects, was 0.868 for the right amygdaloid complex and 0.878 for the right hippocampal formation. In a validity experiment that used phantoms filled with copper sulfate solution, average accuracies of 99.4% for a 2664-ml sphere and 98.3% for a 5-ml cylinder were achieved.
Whole brain volume and total intracranial volume were measured with three-dimensional MRI analyzing software on a graphic workstation (INDIGO II HighImpact, Silicon Graphics, Mountain View, Calif.). The software uses a combination of a gray scale algorithm, an edge-detection algorithm, and some a priori knowledge. With this software, the whole brain was segmented basically by detecting the boundary between the CSF and gray matter, and the calvarium was extracted by detecting the outer surface of the dura mater. The details of this method are described elsewhere together with accuracy data (27, 28). A reliability experiment in which measurement was repeated in 20 randomly selected patients demonstrated that the ICC of the two-operator measurements was greater than 0.999 for both total intracranial volume and whole brain volume. A validity experiment in which measurements of the same samples made by this technique were compared with those made by a semiautomated segmentation method that combined density thresholding with manual tracing (whose accuracy in phantom measurements has been established [23]) demonstrated that the mean difference between the two techniques was 13.7 cm3 for intracranial volume and 3.1 cm3 for brain volume, and that the ICC were 0.951 for intracranial volume and 0.881 for brain volume (27, 28).
Statistical Analysis
To adjust for intersubject variability in cranium size, the volume of each structure was normalized to the total intracranial volume with the covariance method described by Jack et al. (25): normalized volume=observed volume – B × (patient’s total intracranial volume – mean total intracranial volume in normal subjects), where B is the regression coefficient in the normative linear object volume/total intracranial volume relationship. This is considered to be the most accurate method for normalizing absolute hippocampal volume (29).
We used the averaged volumes of the right and left amygdaloid complexes and of the hippocampal formations for the analysis, thereby reducing the number of variables and increasing the reliability of the measurements. To analyze the specific effects of amygdalar and hippocampal volumes on emotional memory, a multiple linear regression partial correlation was used, with total emotional memory score or each of the section A, B, and C scores as the dependent variables and the amygdalar and hippocampal volumes as the independent variables. Possible confounding effects of age, sex, and educational attainment were controlled by entering these variables into the model. To eliminate the effect of whole brain atrophy and the effect of severity of dementia or memory impairment, we thereafter entered normalized whole brain volume and either the Mini-Mental State score, the clinical dementia rating, or the Wechsler Memory Scale total weighted sum score into the model. A partial correlation coefficient was also used to examine the specific effects of amygdalar and hippocampal volumes on Wechsler Memory Scale performance when the confounding factors were controlled. Student’s t test and Pearson’s correlation were used where appropriate. All statistical analyses were carried out with SAS release 6.10 software (SAS Institute, Inc., Cary, N.C.). The level of significance was set at p<0.05.
RESULTS
According to the informants, all patients had recognized the earthquake well and had been involved in everyday talks at home and in town and heard continuous broadcasts about the earthquake. Thirty-one (86.1%) of the patients recalled the earthquake. The mean total emotional memory score was 4.5 (SD=2.5) (maximum possible score=9). Patients achieved mean scores of 2.1 (SD=1.1) on event recall, 2.1 (SD=1.1) on personal memory, and 0.5 (SD=0.7) on general knowledge in the assessment of memory of the earthquake (maximum possible score for each=3). On the Wechsler Memory Scale, the mean weighted score on the verbal subtests was 8.8 (SD=6.6) (normal range in aged people=31–77), the mean weighted score on the visual subtests was 13.5 (SD=7.4) (normal range=32–54), and the mean total weighted sum score was 22.3 (SD=12.7) (normal range=72–122). The event recall scores were significantly correlated with the clinical dementia ratings (r=–0.42, df=34, p=0.01), the Mini-Mental State scores (r=0.52, df=34, p<0.001), and the Wechsler Memory Scale total weighted sum scores (r=0.45, df=34, p=0.005). Similarly, the personal memory scores were significantly correlated with the clinical dementia ratings (r=–0.53, df=34, p<0.001), the Mini-Mental State scores (r=0.53, df=34, p<0.001), and the Wechsler Memory Scale total weighted sum scores (r=0.45, df=34, p=0.006). The general knowledge scores were significantly correlated with the Mini-Mental State scores (r=0.42, df=34, p=0.01) and the Wechsler Memory Scale total weighted sum scores (r=0.38, df=34, p=0.02).
The mean intracranial and whole brain raw volumes were 1445 cm3 (SD=123) and 970 cm3 (SD=96), respectively, in the patients and 1429 cm3 (SD=144) and 1100 cm3 (SD=140), respectively, in the normal subjects. The mean normalized whole brain volume was significantly smaller in the patients (mean=961 cm3, SD=66) than in the normal subjects (mean=1098 cm3, SD=52) (t=11.42, df=61, p<0.001). No significant correlation between normalized whole brain volume and each earthquake-related memory score was noted. The mean amygdalar and hippocampal raw volumes were 1418 cm3 (SD=342) and 2441 cm3 (SD=419), respectively, in the patients and 1798 cm3 (SD=229) and 3154 cm3 (SD=425), respectively, in the normal subjects. The mean normalized volumes of the amygdaloid complex and hippocampal formation in the patients were significantly smaller than those in the normal subjects: for the amygdaloid complex, 1435 cm3 (SD=319) versus 1836 cm3 (SD=174) (t=5.90, df=61, p<0.001); for the hippocampal formation, 2441 cm3 (SD=419) versus 3219 cm3 (SD=313) (t=8.09, df=61, p<0.001).
The correlations between total emotional memory score and normalized volumes of the amygdala and hippocampus are shown in figure 2. Normalized amygdalar volume was significantly correlated with the total emotional memory score, the event recall score, and the personal memory score irrespective of age, sex, and education (table 1). The effects of normalized amygdalar volume on these scores remained significant after the effects of whole brain atrophy and functional/cognitive impairment were controlled. Although a significant correlation between normalized hippocampal volume and the personal memory score was demonstrated in a preliminary analysis, the correlation was no longer significant when the effect of severity of functional impairment was partialed out, suggesting that the correlation is likely to be accounted for mostly by disease severity (table 1). There was no significant anatomical correlate of the general knowledge score (table 1). A marginal correlation was found between normalized hippocampal volume and the Wechsler Memory Scale verbal weighted sum score (partial r=0.31, df=34, p=0.09). When we tested for significant correlations with the Wechsler Memory Scale subtest scores, only the logical memory subtest score was correlated significantly with normalized hippocampal volume (partial r=0.36, df=34, p<0.05).
DISCUSSION
Neurodegenerative changes in Alzheimer’s disease, including neuronal cell loss, are followed by brain atrophy, which is the main gross pathological feature of the disease (14, 15). Studies of autopsied cases of advanced Alzheimer’s disease have also demonstrated that amygdalar atrophy is related to the pathology of the disease (16, 30). They show that the amygdalar atrophy in Alzheimer’s disease is due to the loss of neuronal somata and processes and to the accumulation of neuritic plaque and neurofibrillary tangles, as well as extensive gliosis in discrete amygdalar subnuclei—in particular, the loss of large nerve cells in the magnocellular basolateral amygdalar nuclei group. The morphological deformation relates to intrinsic damage to the amygdalar subnuclei and their reciprocal circuitry with other brain regions. A strong correlation between a decrease in the volume of hippocampal formation subdivisions (the dentate gyrus, area CA4–1, and the subiculum) and a decrease in the total number of neurons in Alzheimer’s disease has been also demonstrated in pathological studies (31, 32). These pathological findings indicate that neuronal loss is a cause of amygdalar and hippocampal volumetric loss and provide a rationale for studies of correlations between MRI volumetry and function in Alzheimer’s disease.
The main finding in this study is that recall performance concerning the earthquake was correlated with the normalized volume of the amygdaloid complex. These correlations were irrespective of age, sex, educational attainment, whole brain atrophy, and severity of dementia or amnesia. In contrast, the normalized volume of the hippocampal formation accounted less for the loss of recollection of emotional events and memories of details of personal episodes. These findings are consistent with those in experimental studies on patients with Urbach-Wiethe disease who had selective bilateral lesions of the amygdala, in which a dysfunction in memorizing emotional materials but not in memorizing neutral materials has been demonstrated (1, 11–13). By using positron emission tomography of glucose metabolism, Cahill et al. (33) demonstrated that the glucose metabolic rate in the right amygdala at encoding was correlated with long-term free recall of emotionally arousing information.
It is noteworthy that nonpersonal factual knowledge of the earthquake, which was very poorly recalled by the patients, was correlated with neither amygdalar volume nor hippocampal volume. On the other hand, recall of details about personal events concerning the earthquake, which was well retained by the patients, was linked to normalized amygdalar volume. This suggests that reinforcement of memory by emotion is limited to high self-reference regarding the material (4). It has been demonstrated that a high affective value and high self-reference of learning material help to improve memory performance, as these factors allow better memory consolidation and better integration of the new material into existing knowledge structures (6). An interaction between emotional involvement and self-reference is plausible, and general knowledge is certainly of low self-reference value and insignificant in an individual’s life (5). Less involvement of emotion in general knowledge would account for the less active participation of the amygdala in general knowledge. Recall of personally experienced time- and place-specific events belongs to episodic memory, while recall of facts that lack particular time and space contexts is involved more in semantic memory (34). Semantic memory is thought to have neural substrates other than the medial temporal memory system, which is critical for episodic memory, e.g., the inferior temporal lobe (35). This may explain the lack of association of general knowledge with hippocampal volume.
The neural substrates of emotional memory in animals have been extensively studied with the use of classical fear conditioning paradigms, suggesting that the amygdala is an essential component in a distributed network of emotional information processing (2, 9, 10). Analogous brain mechanisms in humans have also been proposed (1, 36). Within the amygdala, the lateral nucleus is the sensory interface, which projects to the central nucleus indirectly by way of the basolateral nucleus, and the central nucleus is the motor interface of the amygdala. Afferent inputs from sensory processing areas of the thalamus and cortex mediate emotional learning in situations involving specific sensory cues, whereas learning about the emotional significance of more general, contextual cues involves projections to the amygdala from the hippocampal formation (9). The basolateral, lateral, and central nuclei have been postulated to act as links between sensory information and affective significance and to perform critical functions in acquiring and storing long-term associative memory (2, 9, 10).
Animal studies have indicated that the hippocampus is not essential for emotional memory (9); selective hippocampal lesions impair higher-order contextual associations but not simple conditioned fear associations, while amygdalar lesions disrupt both simple and complex aspects of fear conditioning (37). A study on autobiographical memory in amnesia (7) noted that personal memories associated with a strong emotional flavor are relatively retained in amnesic patients despite severe retrograde amnesia. In experimental settings, Hamann et al. (38, 39) have documented that amnesic patients with hippocampal or diencephalic damage exhibit the normal enhancement of declarative memory associated with emotional material. Our results, however, do not necessarily deny the role of the hippocampus in the emotional memory process, as damage in the hippocampus, which is invariably seen in Alzheimer’s disease (14, 15), might be an indispensable condition for the occurrence of emotional memory deficits. The hippocampus might be involved in aspects of declarative memory processing that are relevant to emotional events. The marginal correlation between hippocampal volume and personal memory score implies that the hippocampus takes part in declarative emotional memory processing. The present study also demonstrated a weak correlation between hippocampal volume and verbal memory. This finding is consistent with the findings in previous MRI volumetric studies (17, 40, 41). Therefore, it is conceivable that the hippocampus is necessary for the declarative memory process, regardless of emotional valence, as a fundamental memory system.
Even with the best MRI volumetric technique currently available (42)—both the amygdala proper and its adjacent cortical regions, including the periamygdaloid and entorhinal cortices, were conjointly measured to maintain a reliable segmentation on MRI—the structures more relevant to emotional memory deficits remain undetermined. Zola-Morgan and his co-workers (43, 44), on the basis of a series of studies of monkeys with discrete lesions in the medial temporal lobe, have demonstrated that the cortical regions surrounding the amygdala, rather than the amygdala proper, contribute to memory impairment. However, since their findings were derived from studies of relatively short-term declarative memory in a relatively nonemotional learning task, they do not conflict with the finding of a role of the amygdala proper in long-term declarative memory of an emotional event.
In conclusion, memory of real-life emotional episodes, like other aspects of episodic memory function, is defective in patients with Alzheimer’s disease, while emotional arousal enhances memory of all aspects of an event except details irrelevant to the individual’s life. Emotional memory deficits in Alzheimer’s disease are related to the intensity of amygdalar damage and less so to the intensity of hippocampal damage. It is likely that the hippocampus is involved mainly in the declarative process of personal memory. This provides further evidence that the amygdala plays a central role in emotional memory in humans.
Questionnaire Regarding the Kobe Earthquake
Section A: Event Recall
1. | Do you know any recent disaster in which many people were killed? | ||||
2. | Great fires broke out and many structures were destroyed by this disaster. Do you remember anything? | ||||
3. | That is the earthquake. Do you remember that? |
Section B: Personal Memory
1. | Were your family members injured by this earthquake? Was your house damaged by this earthquake? | ||||
2. | Were your relatives and friends injured by this earthquake? | ||||
3. | What did you do and how did you feel at the moment of the ground trembling? |
Section C: General Knowledge
1. | Where was the main earthquake-stricken district? (Kobe or south Hogo) | ||||
2. | When did this earthquake happen? (Jan. 17, 1995) | ||||
3. | How many people were killed by this earthquake? (5,000–6,000 people) |
Presented in part at the 122nd annual meeting of the American Neurological Association, San Diego, Sept. 29, 1997. Received April 13, 1998; revision received Aug. 31, 1998; accepted Sept. 4, 1998. From the Department of Clinical Neurosciences and the Department of Neuroimaging Research, Hyogo Institute for Aging Brain and Cognitive Disorders. Address reprint requests to Dr. Mori, Department of Clinical Neurosciences, Hyogo Institute for Aging Brain and Cognitive Disorders, 520 Saisho-ko, Himeji, 670-0981, Japan; [email protected] (e-mail).
![]() |
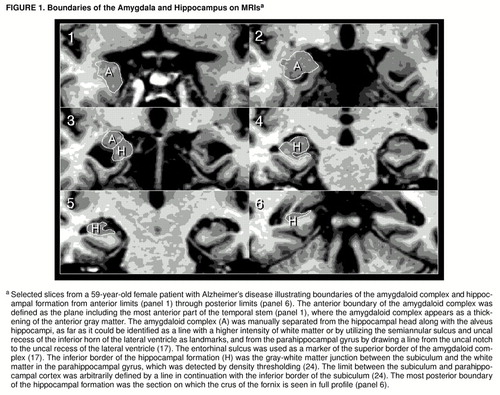
FIGURE 1. Boundaries of the Amygdala and Hippocampus on MRIsa
aSelected slices from a 59-year-old female patient with Alzheimer’s disease illustrating boundaries of the amygdaloid complex and hippocampal formation from anterior limits (panel 1) through posterior limits (panel 6). The anterior boundary of the amygdaloid complex was defined as the plane including the most anterior part of the temporal stem (panel 1), where the amygdaloid complex appears as a thickening of the anterior gray matter. The amygdaloid complex (A) was manually separated from the hippocampal head along with the alveus hippocampi, as far as it could be identified as a line with a higher intensity of white matter or by utilizing the semiannular sulcus and uncal recess of the inferior horn of the lateral ventricle as landmarks, and from the parahippocampal gyrus by drawing a line from the uncal notch to the uncal recess of the lateral ventricle (17). The entorhinal sulcus was used as a marker of the superior border of the amygdaloid complex (17). The inferior border of the hippocampal formation (H) was the gray-white matter junction between the subiculum and the white matter in the parahippocampal gyrus, which was detected by density thresholding (24). The limit between the subiculum and parahippocampal cortex was arbitrarily defined by a line in continuation with the inferior border of the subiculum (24). The most posterior boundary of the hippocampal formation was the section on which the crus of the fornix is seen in full profile (panel 6).
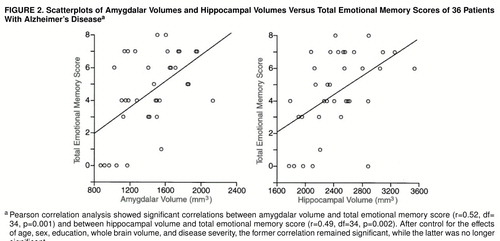
FIGURE 2. Scatterplots of Amygdalar Volumes and Hippocampal Volumes Versus Total Emotional Memory Scores of 36 Patients With Alzheimer’s Diseasea
aPearson correlation analysis showed significant correlations between amygdalar volume and total emotional memory score (r=0.52, df=34, p=0.001) and between hippocampal volume and total emotional memory score (r=0.49, df=34, p=0.002). After control for the effects of age, sex, education, whole brain volume, and disease severity, the former correlation remained significant, while the latter was no longer significant.
1. Cahill L, Babinsky R, Markowitsch HJ, McGaugh JL: The amygdala and emotional memory. Nature 1995; 377:295–296Crossref, Medline, Google Scholar
2. Cahill L, McGaugh JL: Mechanisms of emotional arousal and lasting declarative memory. Trends Neurosci 1998; 21:294–299Crossref, Medline, Google Scholar
3. Brown R, Kulik J: Flashbulb memories. Cognition 1977; 5:73–99Crossref, Google Scholar
4. Bohannon JN III: Flashbulb memories for the space shuttle disaster: a tale of two theories. Cognition 1988; 29:179–196Crossref, Medline, Google Scholar
5. Terr LC, Bloch DA, Michel BA, Shi H, Reinhardt JA, Metayer S: Children’s memories in the wake of Challenger. Am J Psychiatry 1996; 153:618–625Link, Google Scholar
6. Spies K: Einfluss von emotionalem Gehalt und Ichbezug des Lernmaterials auf die Behaltensleistung [Effect of emotional content and self-reference of learning materials on recall performance]. Z Exp Angew Psychol 1994; 41:617–630Medline, Google Scholar
7. Daum I, Flor H, Brodbeck S, Birbaumer N: Autobiographical memory for emotional events in amnesia. Behav Neurol 1996; 9:57–67Crossref, Medline, Google Scholar
8. Cahill L, Prins B, Weber M, McGaugh JL: Beta-adrenergic activation and memory for emotional events. Nature 1994; 371:702–704Crossref, Medline, Google Scholar
9. LeDoux JE: Emotional memory systems in the brain. Behav Brain Res 1993; 58:69–79Crossref, Medline, Google Scholar
10. Davis M: The role of the amygdala in conditioned fear, in The Amygdala: Neurobiological Aspects of Emotion, Memory, and Mental Dysfunction. Edited by Aggleton JP. New York, Wiley-Liss, 1992, pp 255–305Google Scholar
11. Babinsky R, Calabrese P, Durwen HF, Markowitsch HJ, Brechtelsbauer D, Heuser L, Gehlen W: The possible contribution of the amygdala to memory. Behav Neurol 1993; 6:167–170Crossref, Medline, Google Scholar
12. Markowitsch HJ, Calabrese P, W�rker M, Durwen HF, Kessler J, Babinsky R, Brechtelsbauer D, Heuser L, Gehlen W: The amygdala’s contribution to memory: a study on two patients with Urbach-Wiethe disease. Neuroreport 1994; 5:1349–1352Medline, Google Scholar
13. Adolphs R, Cahill L, Schul R, Babinsky R: Impaired declarative memory for emotional material following bilateral amygdala damage in humans. Learning and Memory 1997; 4:291–300Crossref, Medline, Google Scholar
14. Hyman BT, Van Hoesen GW, Damasio AR, Barnes CL: Alzheimer’s disease: cell-specific pathology isolates the hippocampal formation. Science 1984; 225:1168–1170Crossref, Medline, Google Scholar
15. Hyman BT, Van Hoesen GW, Damasio AR: Memory-related neural systems in Alzheimer’s disease: an anatomic study. Neurology 1990; 40:1721–1730Crossref, Medline, Google Scholar
16. Scott SA, DeKosky ST, Sparks DL, Knox CA, Scheff SW: Amygdala cell loss and atrophy in Alzheimer’s disease. Ann Neurol 1992; 32:555–563Crossref, Medline, Google Scholar
17. Mori E, Yoneda Y, Yamashita H, Hirono N, Ikeda M, Yamadori A: Medial temporal structures relate to memory impairment in Alzheimer’s disease: an MRI volumetric study. J Neurol Neurosurg Psychiatry 1997; 63:214–221Crossref, Medline, Google Scholar
18. Ikeda M, Mori E, Hirono N, Imamura T, Shimomura T, Ikejiri Y, Yamashita H: Amnestic Alzheimer patients remembered the Kobe earthquake. Br J Psychiatry 1998; 172:425–428Crossref, Medline, Google Scholar
19. McKhann G, Drachman D, Folstein M, Katzman R, Price D, Stadlan EM: Clinical diagnosis of Alzheimer"s disease: report of the NINCDS-ADRDA Work Group under the auspices of the Department of Health and Human Services Task Force on Alzheimer"s Disease. Neurology 1984; 34:939–944Crossref, Medline, Google Scholar
20. Folstein MF, Folstein SE, McHugh PR: “Mini-Mental State”: a practical method for grading the cognitive state of patients for the clinician. J Psychiatr Res 1975; 12:189–198Crossref, Medline, Google Scholar
21. Morris JC: The Clinical Dementia Rating (CDR): current version and scoring rules. Neurology 1993; 43:2412–2414Crossref, Medline, Google Scholar
22. Wechsler D: Wechsler Memory Scale—Revised. San Antonio, Psychological Corp, 1981Google Scholar
23. Mori E, Hirono N, Yamashita H, Imamura T, Ikejiri Y, Ikeda M, Kitagaki H, Shimomura T, Yoneda Y: Premorbid brain size as a determinant of reserve capacity against intellectual decline in Alzheimer’s disease. Am J Psychiatry 1997; 154:18–24Link, Google Scholar
24. Hashimoto M, Kitagaki H, Imamura T, Hirono N, Tanimukai S, Hanihara T, Mori E: Medial temporal and whole brain atrophy in dementia with Lewy bodies: a volumetric MRI study. Neurology 1998; 51:357–362Crossref, Medline, Google Scholar
25. Jack CR, Twomey CK, Zinsmeister AR, Sharborough FW, Petersen R, Cascino GD: Anterior temporal lobes and hippocampal formations: normative volumetric measurements from MR images in young adults. Radiology 1989; 172:549–554Crossref, Medline, Google Scholar
26. Yoneda Y, Mori E, Yamadori A, Yamashita H: MRI volumetry of medial temporal lobe structures in amnesia following herpes simplex encephalitis. Eur Neurol 1994; 34:243–252Crossref, Medline, Google Scholar
27. Yasuda M, Mori E, Kitagaki H, Yamashita H, Hirono N, Shimada K, Maeda K, Tanaka C: Apolipoprotein E ε4 allele and whole brain atrophy in late-onset Alzheimer’s disease. Am J Psychiatry 1998; 155:779–784Abstract, Google Scholar
28. Kitagaki H, Mori E, Yamaji S, Ishii K, Hirono N, Kobashi S, Hata Y: Frontotemporal dementia and Alzheimer disease: evaluation of cortical atrophy with automated hemispheric surface display generated with MR images. Radiology 1998; 208:431–439Crossref, Medline, Google Scholar
29. Free SL, Bergin PS, Gish DR, Cook MJ, Shorvon SD, Stevens JM: Methods for normalization of hippocampal volumes measured with MR. AJNR Am J Neuroradiol 1995; 16:637–643Medline, Google Scholar
30. Scott SA, DeKosky ST, Scheff SW: Volumetric atrophy of the amygdala in Alzheimer’s disease: quantitative serial reconstruction. Neurology 1991; 41:351–356Crossref, Medline, Google Scholar
31. Bobinski M, Wegiel J, Wisniewski HM, Tarnawski M, Bobinski M, Reisberg B, De Leon MJ, Miller DC: Neurofibrillary pathology—correlation with hippocampal formation atrophy in Alzheimer disease. Neurobiol Aging 1996; 17:909–919Medline, Google Scholar
32. Simic G, Kostovic I, Winblad B, Bogdanovic N: Volume and number of neurons of the human hippocampal formation in normal aging and Alzheimer’s disease. J Comp Neurol 1997; 379:482–494Crossref, Medline, Google Scholar
33. Cahill L, Haier RJ, Fallon J, Alkire M, Tang C, Keator D, Wu J, McGaugh JL: Amygdala activity at encoding correlated with long-term, free recall of emotional information. Proc Natl Acad Sci USA 1996; 93:8016–8021Crossref, Medline, Google Scholar
34. Squire LR: Memory and Brain. New York, Oxford University Press, 1987Google Scholar
35. Garrard P, Perry R, Hodges JR: Disorders of semantic memory. J Neurol Neurosurg Psychiatry 1997; 62:431–435Crossref, Medline, Google Scholar
36. LaBar KS, Gatenby JC, Gore JC, LeDoux JE, Phelps EA: Human amygdala activation during conditioned fear acquisition and extinction: a mixed-trial fMRI study. Neuron 1998; 20:937–945Crossref, Medline, Google Scholar
37. Kim JJ, Fanselow MS: Modality-specific retrograde amnesia of fear. Science 1992; 256:675–677Crossref, Medline, Google Scholar
38. Hamann SB, Cahill L, McGaugh J, Squire LR: Intact enhancement of declarative memory for emotional material in amnesia. Learning and Memory 1997; 4:301–309Crossref, Medline, Google Scholar
39. Hamann SB, Cahill L, Squire LR: Emotional perception and memory in amnesia. Neuropsychology 1997; 11:104–113Crossref, Medline, Google Scholar
40. Deweer B, Leh�ricy S, Pillon B, Baulac M, Chiras J, Marsault C, Agid Y, Dubois B: Memory disorders in probable Alzheimer’s disease: the role of hippocampal atrophy as shown with MRI. J Neurol Neurosurg Psychiatry 1995; 58:590–597Crossref, Medline, Google Scholar
41. Laakso MP, Soininen H, Partanen K, Helkala EL, Hartikainen P, Vainio P, Hallikainen M, Hanninen T, Riekkinen PJ Sr: Volumes of hippocampus, amygdala and frontal lobes in the MRI-based diagnosis of early Alzheimer’s disease: correlation with memory functions. J Neural Transm Park Dis Dement Sect 1995; 9:73–86Crossref, Medline, Google Scholar
42. Jack CR Jr: MRI-based hippocampal volume measurements in epilepsy. Epilepsia 1994; 35(suppl 6):21–29Google Scholar
43. Zola-Morgan S, Squire LR, Amaral DG: Lesions of the amygdala that spare adjacent cortical regions do not impair memory or exacerbate the impairment following lesions of the hippocampal formation. J Neurosci 1989; 9:1922–1936Crossref, Medline, Google Scholar
44. Zola-Morgan S, Squire LR, Amaral DG, Suzuki WA: Lesions of perirhinal and parahippocampal cortex that spare the amygdala and hippocampal formation produce severe memory impairment. J Neurosci 1989; 9:4355–4370Crossref, Medline, Google Scholar