Amphetamine-Induced Dopamine Release: Markedly Blunted in Cocaine Dependence and Predictive of the Choice to Self-Administer Cocaine
Abstract
Objective: Dopamine is an important mediator of the reinforcing effects of cocaine, and alterations in dopamine function might be involved in cocaine dependence. The goals of the present study were to characterize pre- and postsynaptic dopamine function in recently detoxified cocaine-dependent subjects. Specifically, dopamine response to an acute amphetamine challenge was assessed in striatal subregions in cocaine-dependent and healthy comparison participants using positron emission tomography (PET). Furthermore, the relationship between this dopamine response and the choice to self-administer cocaine in a laboratory model of relapse was investigated. Method: Twenty-four cocaine-dependent participants and 24 matched healthy subjects underwent [ 11 C]raclopride scans under a baseline condition and following intravenous amphetamine administration (0.3 mg/kg). Cocaine-dependent participants also completed cocaine self-administration sessions in which a priming dose of cocaine was followed by the choice to either self-administer subsequent cocaine doses or receive a monetary reward. Results: Cocaine dependence was associated with a marked reduction in amphetamine-induced dopamine release in each of the functional subregions of the striatum (limbic striatum: –1.2% in cocaine-dependent participants versus –12.4% in healthy subjects; associative striatum: –2.6% versus –6.7%, respectively; sensorimotor striatum: –4.3% versus –14.1%). Blunted dopamine transmission in the ventral striatum and anterior caudate was predictive of the choice for cocaine over money. Conclusions: Cocaine dependence is associated with impairment of dopamine function, and this impairment appears to play a critical role in relapse.
Preclinical models of cocaine dependence have shown that maladaptive changes in dopamine transmission of the striatum play a critical role in this disorder. However, the significance of these changes has yet to be fully understood in humans. Positron emission tomography (PET) and the dopamine type 2 and 3 receptor (D 2/3 ) radiolabeled antagonist [ 11 C]raclopride can be used to measure changes in extracellular dopamine in the human brain. The administration of a psychostimulant is associated with an increase in endogenous dopamine and a reduction in the binding of [ 11 C]raclopride. Thus the comparison of pre- and postamphetamine scans provides a measure of in vivo dopamine release (1) .
A previous study by Volkow et al. (2) performed in human cocaine-dependent volunteers demonstrated a reduction in methylphenidate-induced dopamine release in the striatum, consistent with a loss of presynaptic dopamine function. This study was performed measuring the striatum as a whole, so the first goal of the present study was to replicate this finding with a high-resolution PET camera that allows investigation of dopamine transmission in limbic, associative, and sensorimotor subdivisions of the striatum.
The clinical significance of this deficit of dopamine transmission was also investigated using a behavioral model of relapse. In animals, a response-independent (“priming”) dose of cocaine has been shown to reinstate cocaine self-administration (3 – 5) . Using a similar laboratory model, we can investigate the effect of a priming dose of cocaine on the choice to self-administer cocaine in human participants (6 , 7) . Thus, the second goal of this study was to investigate the association between deficits in presynaptic dopamine and the choice for cocaine in this model of relapse.
The hypotheses of this study were 1) cocaine dependence would be associated with a reduction in amphetamine-induced dopamine release across the subdivisions of the striatum, and the decrease would be most pronounced in the limbic striatum, and 2) the loss of amphetamine-induced dopamine release in the limbic striatum would be associated with the choice to self-administer cocaine.
Method
The study was approved by the institutional review board of the New York State Psychiatric Institute. All participants provided written informed consent. The cocaine-dependent participants fulfilled DSM-IV criteria for cocaine dependence with no other axis I diagnosis. Cocaine-dependent participants were not treatment seeking and were admitted to the Irving Center for Clinical Research. PET scans were performed after a minimum of 14 days of abstinence and cocaine self-administration sessions occurred after the PET scans. Healthy comparison participants had no DSM-IV axis I disorder and participated as outpatients. Nicotine dependence was acceptable for both groups.
PET Scan Analysis
[ 11 C]Raclopride was administered as a bolus with constant infusion, and the PET scans were acquired on the ECAT EXACT HR+ (Siemens/CTI, Knoxville, Tenn.) in 3-dimensional mode as eight frames of 5 minutes each (obtained from 40–80 minutes) as previously described (8) . All participants underwent two scans with [ 11 C]raclopride: baseline and following intravenous administration of amphetamine (0.3 mg/kg). An MRI (GE 1.5-T Signa Horizon) was acquired for each participant for identification of the regions of interest (8) . Four venous samples were analyzed to obtain the plasma concentration of unmetabolized [ 11 C]raclopride (Ci/ml) as previously described (8) . [ 11 C]Raclopride clearance (liters/hour), free fraction (f 1 ), and plasma amphetamine levels were measured as previously described (9 , 10) .
Following amphetamine administration, vital signs were acquired at baseline and at regular intervals as previously described (11) . The area under the curve of the change from baseline was calculated for systolic blood pressure, diastolic blood pressure, and heart rate. The subjective response to amphetamine was evaluated using a simplified version of the Amphetamine Interview Rating Scale, in which euphoria, energy, restlessness, and anxiety were rated on a scale of 1 (least) to 10 (most) as previously described (11) .
PET Outcome Measures
D 2/3 receptor availability was estimated for the pre- and postamphetamine scans using two outcome measures: [ 11 C]raclopride binding potential (ml/g), defined as:
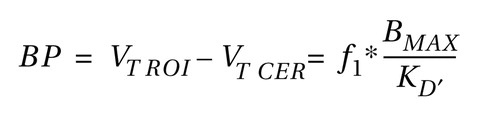
and the specific-to-nonspecific partition coefficient (V 3 ′′, unitless), defined as:
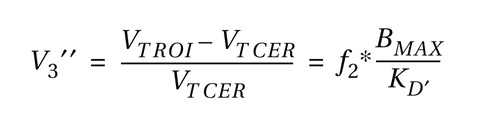
where V T is the tissue distribution volume for the regions of interest (V T ROI ) and cerebellum (V T CER ), f 2 is the free fraction in the nonspecific distribution volume, B max is the concentration of D 2/3 receptors, and K′ D is the in vivo equilibrium dissociation constant of the radiotracer in the presence of dopamine (12) .
The reduction in D 2 receptor availability following amphetamine (ΔV 3 ′′) was calculated as:

Region of Interest Analysis
Image analysis was performed in MEDx (Sensor Systems, Inc., Sterling, Va.). The regions of interest were drawn on each subject’s MRI, and PET-to-MRI registration was performed as previously described (8) . The striatum was divided as previously described (11) into the caudate, putamen, and ventral striatum. The caudate and putamen were further subdivided along their rostral-caudal axis using the anterior commissure to derive the following regions of interest: 1) precommissural dorsal caudate, 2) precommissural dorsal putamen, 3) the postcommissural caudate, and 4) the postcommissural putamen. These regions of interest were then classified into the three following functional subdivisions: 1) the limbic striatum , which receives input from limbic structures and includes the nucleus accumbens (also referred to as the ventral striatum); 2) the associative striatum , which is largely involved in cognition and includes the precommissural dorsal caudate, precommissural dorsal putamen, and postcommissural caudate; and 3) the sensorimotor striatum , which mostly receives input from motor and premotor areas and includes the postcommissural putamen.
The outcome measures for the associative striatum were calculated from the weighted average of the precommissural dorsal caudate, postcommissural caudate, and precommissural dorsal putamen, while the outcome measures for the striatum were calculated as the weighted average of all five regions of interest. Because of limitations in PET camera resolution, the activity measured in a given region of interest includes activity from adjacent regions, which result in error due to partial volume effects. In order to address this error, partial volume effect correction was performed as described previously (13) and in the data supplement that accompanies the online version of this article.
Voxel-Wise Analysis
V 3 ′′ images were formed from the MRI-coregistered PET data and derived from the equation: (mean PET voxel value over scanning period/mean cerebellum activity over scanning period) – 1. The individual MRI images were normalized to the MNI TI template with nonlinear warping using Statistical Parametric Mapping 1999 (SPM99) (14) . The transformation parameters were then applied to the V 3 ′′ images (pre- and postamphetamine) to obtain a set of spatially normalized V 3 ′′ images. ΔV 3 ′′ images were then formed as 1 – (amphetamine condition/control condition). Prior to the statistical analysis, data were smoothed with a 6-mm isotropic Gaussian kernel.
Self-Administration Sessions
The cocaine-dependent participants completed two types of cocaine self-administration sessions: sample sessions and choice sessions. In the sample sessions, the participants self-administered a single dose of smoked cocaine (three sessions of 0, 6, or 12 mg of cocaine) and were asked to rate the subjective effects as previously described (15) . Visual analogue scales were used to measure the subjective effects of “good drug effect,” “high,” and “stimulated” which were grouped into the positive effects cluster (6) .
Each cocaine-dependent participant also underwent three cocaine self-administration sessions with 0 mg, 6 mg, and 12 mg doses, as previously described (6 , 7) . Each session began with a response-independent or “priming” dose of cocaine. Following this dose, participants were given five choices between smoking the same dose of cocaine or receiving a $5.00 merchandise voucher redeemable at local stores and paid upon discharge. The outcome measure for the choice sessions was the number of times a dose of cocaine was chosen over the voucher (range from 0 to 5).
Statistical Analysis
Group demographic comparisons were performed with unpaired t tests. Group differences in pre- and postamphetamine D 2/3 receptor availability as well as differences in reduction in D 2/3 receptor availability following amphetamine were analyzed with a repeated measures ANOVA, with the region of interest as the repeated measure and diagnostic group as the cofactor. The use of binding potential for between-group comparisons assumes that f 1 is not significantly different between groups, whereas the use of V 3 ′′ assumes that f 2 is not significantly different between groups (12) . For the between-group analyses, f 1 , binding potential, and V 3 ′′ were measured to assess the validity of these assumptions. For the within-subject analysis, V 3 ′′ provides a more robust outcome measure (16) , so ΔV 3 ′′ was chosen a priori to assess the amphetamine-induced reduction in D 2/3 receptor availability. For voxelwise analysis, the analysis was restricted to voxels in which V 3 ′′ ≥ 1 in the baseline condition. Multiple comparison corrections were performed according to the Gaussian random field model employed in the SPM99 software.
Results
Twenty-four cocaine-dependent subjects (19 men and five women, mean age=39 years [SD=3]) and 24 healthy comparison subjects (19 men and five women, mean age= 38 years [SD=5]) were enrolled in this study. Seventeen of the subjects from each group were included in a previous study that included only the baseline scan with [ 11 C]raclopride (7) . Subjects were matched for ethnicity (healthy group: African-American [N=12], Caucasian [N=7], Hispanic [N=5]; cocaine-dependent group: African-American [N=16], Caucasian [N=4], Hispanic [N=4]) and cigarette smoking (the comparison group smoked on average 12 cigarettes/day [SD=6] and included six nonsmokers and three ex-smokers; the cocaine-dependent group smoked on average 11 cigarettes/day [SD=4] and included four nonsmokers and three ex-smokers). The cocaine-dependent subjects reported smoking crack cocaine an average of 16.1 years (SD=4.4) and were spending $280 weekly (SD=108).
PET Scan Parameters
Table 1 shows the [ 11 C]raclopride scan parameters for the two groups. The volumes of the regions of interest did not differ between the two groups (all p>0.20). Although there was no significant difference for cerebellar tissue distribution volume between the two groups before or after amphetamine, there was a small but significant decrease after amphetamine when the data for the two groups was pooled (p<0.001, paired t test) with no significant difference in f 2 (p=0.53).
Baseline D 2/3 Receptor Availability
Repeated-measures ANOVA revealed that cocaine dependence was associated with significantly lower baseline [ 11 C]raclopride binding potential (region factor: p<0.001; group factor: p=0.014; group-by-region interaction: p=0.009) and V 3 ′′ (region factor: p<0.001; group factor: p=0.001; group-by-region interaction: p=0.0015), as shown in Table 2 . These decreases in baseline binding potential and V 3 ” were similar for each of the regions of interest except for the postcommissural caudate. Data corrected for the partial volume effects provided the same results and are presented in the supplement that accompanies the online version of this article.
Amphetamine-Induced Reduction in D 2/3 Receptor Availability
Repeated-measures ANOVA revealed that cocaine dependence was associated with a blunted effect of amphetamine on [ 11 C]raclopride V 3 ′′ (region factor: p<0.0001; group factor: p=0.0009; group-by-region interaction: p<0.0001 [ Table 3 ]). In the caudate, ΔV 3 ′′ was reduced in the cocaine-dependent participants relative to the comparison subjects, but this difference did not reach significance in either the precommissural dorsal caudate or postcommissural caudate. Similar results were seen following correction for partial volume effects (supplemental data). Post hoc examination showed that dopamine release was blunted to a greater degree in the limbic striatum in cocaine-dependent relative to healthy subjects compared with each of the regions of the associative striatum (all p<0.04), but that there was no difference between the ventral striatum and posterior putamen (p=0.20).
Cocaine dependence was associated with a lower subjective rating of euphoria in response to amphetamine relative to healthy subjects (1.7[SD=60.5] versus 38.1 [SD=63.8], respectively; p<0.04), with no between-group differences in restlessness, anxiety, or energy. No association was seen between ΔV 3 ′′ and the subjective effects of amphetamine for both the healthy controls and cocaine dependent subjects. As shown in Table 4 , no difference in amphetamine-induced changes in vital signs was seen between the two groups.
Self-Administration Session Results
All participants completed the sample sessions, but only 23 of the 24 cocaine-dependent participants completed the choice sessions. In the sample sessions, the area under the curve of the positive effects cluster of the 12-mg dose (323 [SD=633]) was higher than that of the 0-mg dose (140 [SD=449]) and 6-mg dose (155 [SD=427]) (p≤0.05 for both comparisons). The 6-mg dose did not differ significantly from the 0-mg dose, so the positive effects of the 12-mg dose were selected for comparison with the scan data.
In the choice sessions, the 0-mg dose was chosen an average of 0.35 times (SD=1.07) out of a possible five choices, the 6-mg dose was chosen 1.65 times (SD=1.67), and the 12-mg dose was chosen 3.26 times (SD=1.63). Low doses of cocaine were used to ensure enough variability between participants to allow comparison with the [ 11 C]raclopride data. The coefficient of variation was greater for the 6-mg dose than the 12-mg dose (1.01 versus 0.50, respectively), so the 6-mg dose was chosen a priori for comparison with the PET data.
Relationship Between PET Data and Cocaine Self-Administration
No significant relationship was observed between baseline V 3 ′′ and either the positive effects of 12 mg cocaine or the choice for 6 mg cocaine with either the voxel-wise analysis or the region-of-interest analysis (all p>0.12).
In the voxel-wise analysis, no significant clusters were seen for the correlation between ΔV 3 ′′ and the positive effects of 12 mg cocaine. However, bilateral clusters were observed in the ventral striatum where the relationship between ΔV 3 ′′ and the choice for the 6-mg dose were significant (p=0.001, cluster level analysis, left and right) as shown in Figure 1 . The MNI coordinates of the peak t values in these clusters were (–14, 10, –6) (right) and (18, 8, –8) (left), which translate to Talairach coordinates (–13.9, 9.4, –5.5) and (17.8, 7.4, –7.1) respectively, and correspond to the location of the ventral striatum (nucleus accumbens, ventral putamen, and ventral caudate) in the brain atlas of Talairach and Tournoux (17) . No significant clusters were seen outside the ventral striatum.
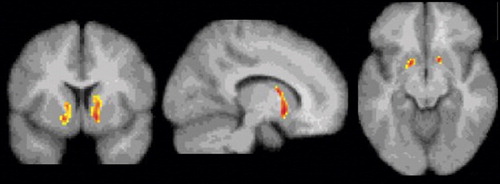
a Using SPM99, T maps with multiple comparison correction were generated to measure the significance of the correlation between ΔV 3 ′′ and the number of cocaine choices (N=23, df=21). The display threshold was set at p≤0.01, uncorrected. A significant bilateral negative correlation was seen in the ventral striatum, showing that cocaine-dependent participants with the lowest dopamine transmission were those who were more likely to choose cocaine over an alternative reinforcer.
In the region-of-interest analysis, no significant relationship was observed between ΔV 3 ′′ and the positive effects of 12 mg cocaine (all p>0.15). A significant negative relationship was observed between ΔV 3 ′′ and the choice for 6 mg cocaine in the ventral striatum (r=–0.55, p=0.007), postcommissural putamen (r=–0.45, p=0.03), and precommissural dorsal caudate (r=–0.54, p=0.007). After correction for multiple comparisons, only the correlation seen in the ventral striatum and precommissural dorsal caudate was significant. Similar results were seen for the association between ΔV 3 ′′ and the 12-mg dose choice (ventral striatum: r=–0.44, p=0.03; precommissural dorsal putamen: r=–0.41, p=0.05; postcommissural putamen: r=–0.43, p=0.04; precommissural dorsal caudate: r=–0.40, p=0.06; postcommissural caudate: r=–0.25, p=0.25).
Discussion
The results of this study demonstrate that cocaine dependence is associated with a reduction in D 2/3 receptor availability and a decrease in amphetamine-induced dopamine release in the ventral striatum and putamen. In the cocaine-dependent volunteers, the decrease in amphetamine-induced dopamine release correlated with the choice for cocaine in the self-administration sessions, such that participants with the greater impairment in presynaptic dopamine in the anterior caudate and ventral striatum were the more likely to choose cocaine over an alternative reinforcer. Insofar as the self-administration sessions provide a valid predictor of relapse, these findings suggest that impairment in presynaptic dopamine function plays a critical role in maintaining the habitual, maladaptive patterns of behavior that are indicative of addiction.
Baseline D 2/3 Receptor Availability
In this expanded dataset, we observed our previously reported finding that cocaine dependence is associated with reduction in D 2/3 receptor availability (7) , which is in agreement with the previous studies of Volkow et al. (2 , 18) . Although V 3 ′′ was decreased in the caudate posterior to the anterior commissure (postcommissural caudate) of the cocaine-dependent participants compared with the healthy subjects, this difference did not reach significance even after correction for partial volume effects, presumably because of the noise associated with this narrow region. We also replicated our previous finding showing that baseline D 2 receptor availability was not predictive of the choice to self-administer cocaine over an alternative reinforcer (7) .
Dopamine Presynaptic Transmission
In contrast to the modest magnitude of the decrease in D 2/3 receptor availability, the deficit in presynaptic dopamine function in the cocaine-dependent participants was severe. Relative to healthy subjects, cocaine-dependent participants showed a 90%, 61%, and 70% reduction in ΔV 3 ′′ in limbic, associative, and sensorimotor regions, respectively. This reduction was significantly greater in the limbic striatum than in the associative striatum, but not significantly different from the sensorimotor striatum. In other words, we did not support our hypothesis that cocaine dependence would be associated with a greater reduction in the limbic striatum. Instead, we demonstrated a similar decrease in the limbic and sensorimotor striatum, suggesting that cocaine dependence is associated with a prominent motor component in addition to changes in limbic dopamine transmission.
With respect to the individual regions of interest, there was a significant group difference in the ventral striatum and putamen but not the caudate. This might be related to the fact that the effect of amphetamine on [ 11 C]raclopride V 3 ′′ is low in the caudate of healthy subjects (11 , 13 , 19) . The blunted dopamine response to amphetamine in cocaine-dependent participants could not be attributed to pharmacokinetic differences in amphetamine, since there was no difference in amphetamine plasma levels or vital signs between the two groups. Nevertheless, the decrease in amphetamine-induced dopamine release in cocaine abusers in this study is in agreement with Volkow et al. (2) , who showed a similar reduction in [ 11 C]raclopride displacement in the striatum as a whole following intravenous methylphenidate (0.5 mg/kg).
The results of these imaging studies are in contrast to preclinical studies demonstrating sensitization of the dopamine response in the striatum to stimulants in laboratory animals exposed to cocaine (20 – 22) . In order for sensitization to occur, the animal must undergo exposure to cocaine followed by a period of abstinence. Given these findings, it would be expected that chronic cocaine abusers administered a psychostimulant would show an excess of dopamine release rather than a blunted effect. The study of Volkow et al. (2) and our study were performed on participants who had been abusing cocaine for prolonged periods of time and the scans were performed following a period of abstinence (3–6 weeks in the study of Volkow et al. and 14 days in our study), such that dopamine sensitization should have been elicited. Therefore, these studies suggest that dopamine sensitization may not be present in humans who have been exposed to cocaine for several years.
The decrease in dopamine transmission is consistent with the observations that, in rodents, chronic cocaine exposure is associated with a reduction in axonal transport of dopamine to the striatum as well as a decrease in cocaine-induced dopamine release (23 – 26) . Postmortem studies in humans have shown that cocaine dependence is associated with a decrease in the levels of vesicular monoamine transporter type 2, which may represent a decrease in or injury to the midbrain dopamine neurons (27 , 28) . Furthermore, Wu et al. (29) demonstrated a reduction in [ 18 F]6-FDOPA uptake, which provides a measure of dopamine synthesis in the axon terminals of the striatum in abstinent cocaine abusers. Together, these studies support the hypothesis that cocaine dependence is associated with a loss of presynaptic dopamine transmission.
In this context, it is important to mention that Volkow et al. (2) reported a greater response to methylphenidate in the thalamus in the cocaine-dependent volunteers (29% versus no effect in healthy subjects), suggesting sensitization may occur in this brain region. Based on the finding of Volkow et al., we performed an exploratory analysis of this region but the difference between cocaine-dependent and healthy subjects did not reach significance (ΔV 3 ′′=5.2% [SD=18.3%] and 3.2% [SD=16.8%], respectively; p=0.70). No correlation was seen between dopamine release in the thalamus and the choice for cocaine, positive effects of cocaine, or subjective effects of amphetamine.
Dopamine Transmission and the Choice to Self-Administer Cocaine
The deficit in dopamine function in the ventral striatum and anterior caudate was predictive of the choice for cocaine over a monetary reward in the laboratory setting. Insofar as these sessions serve as a model of relapse, this finding suggests that cocaine-dependent subjects who are the most vulnerable to relapse are those with the lowest presynaptic dopamine function. This finding was limited to the ventral striatum in the voxel-based analysis. However, a region of interest analysis of ΔV 3 ′′ and the choice for cocaine demonstrated a significant negative association in the caudate rostral to the anterior commissure in addition to the ventral striatum. It should be noted that amphetamine, rather than cocaine, was chosen for challenge because amphetamine can be administered to healthy subjects and since the correlation between dopamine and radiotracer displacement has been established in microdialysis studies on nonhuman primates (30 , 31) . Nevertheless, future studies using a cocaine challenge would be of interest, since cocaine and amphetamine have different mechanisms of action.
The importance of striatal dopamine transmission in brain reward mechanisms has been established through numerous studies (32) . In nonhuman primates, dopamine has been described as encoding a “reward prediction signal” which plays a critical role in discriminating between competing rewards (33) . Dopamine has also been described as mediating the “incentive salience” of a reward (34) or mediating the behavioral economics of reward (35) . These studies suggest that dopamine serves to modify goal directed behavior, as originally hypothesized by Bindra (36) .
Thus, dopamine may provide the signal that emphasizes a particular reward, even at the expense of a competing reward. This hypothesis is in agreement with the present study. In the self-administration sessions, participants were given the choice between low-dose cocaine and a voucher worth $5, both of which serve as reinforcers. However, the doses of cocaine had a street value of less than $5, so that the choice options were weighted toward the money. The failure of the dopamine-depleted cocaine-dependent participants to alter their behavior suggests that these individuals have the greatest deficit in their ability to shift between reward-directed behaviors. We propose that, in this setting, dopamine transmission is critical for making the shift from a habitual behavior that carries a lesser reward (the choice for cocaine) to a more adaptive behavior that is associated with a greater (albeit delayed) reward (the choice for money). The loss of dopamine may represent a loss in the ability to shift from the habitual behavior to another behavior, even one that is associated with a reward. Ultimately, this loss of dopamine may underlie the inability of the individuals with refractory cocaine addiction to respond to therapeutic attempts to substitute more adaptive rewards for habitual cocaine use. Future studies are needed to address this question.
1. Laruelle M: Imaging synaptic neurotransmission with in vivo binding competition techniques: a critical review. J Cereb Blood Flow Metab 2000; 20:423–451Google Scholar
2. Volkow ND, Wang GJ, Fowler JS, Logan J, Gatley SJ, Hitzemann R, Chen AD, Dewey SL, Pappas N: Decreased striatal dopaminergic responsiveness in detoxified cocaine-dependent subjects. Nature 1997; 386:830–833Google Scholar
3. Self DW, Barnhart WJ, Lehman DA, Nestler EJ: Opposite modulation of cocaine-seeking behavior by D1- and D2-like dopamine receptor agonists. Science 1996; 271:1586–1589Google Scholar
4. Khroyan TV, Barrett-Larimore RL, Rowlett JK, Spealman RD: Dopamine D1- and D2-like receptor mechanisms in relapse to cocaine-seeking behavior: effects of selective antagonists and agonists. J Pharmacol Exp Ther 2000; 294:680–687Google Scholar
5. Shaham Y, Shalev U, Lu L, De Wit H, Stewart J: The reinstatement model of drug relapse: history, methodology and major findings. Psychopharmacology (Berl) 2003; 168(1–2):3–20Google Scholar
6. Foltin RW, Ward AS, Collins ED, Haney M, Hart CL, Fischman MW: The effects of venlafaxine on the subjective, reinforcing, and cardiovascular effects of cocaine in opioid-dependent and non-opioid-dependent humans. Exp Clin Psychopharmacol 2003; 11:123–130Google Scholar
7. Martinez D, Broft A, Foltin RW, Slifstein M, Hwang DR, Huang Y, Perez A, Frankle WG, Cooper T, Kleber HD, Fischman MW, Laruelle M: Cocaine dependence and D2 receptor availability in the functional subdivisions of the striatum: relationship with cocaine-seeking behavior. Neuropsychopharmacology 2004; 29:1190–1202Google Scholar
8. Mawlawi O, Martinez D, Slifstein M, Broft A, Chatterjee R, Hwang DR, Simpson N, Ngo K, Van Heertum R, Laruelle M: Imaging human mesolimbic dopamine transmission with PET, I: accuracy and precision of D2 parameter measurements in the ventral striatum. J Cereb Blood Flow Metab 2001; 21:1034–1057Google Scholar
9. Gandelman MS, Baldwin RM, Zoghbi SS, Zea-Ponce Y, Innis RB: Evaluation of ultrafiltration for the free fraction determination of single photon emission computerized tomography (SPECT) radiotracers: β-CIT, IBF and iomazenil. J Pharmaceutical Sci 1994; 83:1014–1019Google Scholar
10. Reimer ML, Mamer OA, Zavitsanos AP, Siddiqui AW, Dadgar D: Determination of amphetamine, methamphetamine and desmethyldeprenyl in human plasma by gas chromatography/negative ion chemical ionization mass spectrometry. Biol Mass Spectrom 1993; 22:235–242Google Scholar
11. Martinez D, Slifstein M, Broft A, Mawlawi O, Hwang DR, Huang Y, Cooper T, Kegeles L, Zarahn E, Abi-Dargham A, Haber SN, Laruelle M: Imaging human mesolimbic dopamine transmission with positron emission tomography, part II: amphetamine-induced dopamine release in the functional subdivisions of the striatum. J Cereb Blood Flow Metab 2003; 23:285–300Google Scholar
12. Slifstein M, Laruelle M: Models and methods for derivation of in vivo neuroreceptor parameters with PET and SPECT reversible radiotracers. Nucl Med Biol 2001; 28:595–608Google Scholar
13. Mawlawi O, Martinez D, Slifstein M, Broft A, Chatterjee R, Hwang DR, Huang Y, Simpson N, Ngo K, Van Heertum R, Laruelle M: Imaging human mesolimbic dopamine transmission with positron emission tomography, I: accuracy and precision of D2 receptor parameter measurements in ventral striatum. J Cereb Blood Flow Metab 2001; 21:1034–1057Google Scholar
14. Friston KJ, Holmes AP, Worsley KJ, Poline J-P, Frith CD, Frakowiak RSJ: Statistical parametric maps in functional imaging: a general linear approach. Hum Brain Mapp 1995; 2:189–210Google Scholar
15. Evans SM, Haney M, Foltin RW: The effects of smoked cocaine during the follicular and luteal phases of the menstrual cycle in women. Psychopharmacology (Berl) 2002; 159:397–406Google Scholar
16. Laruelle M, Abi-Dargham A, van Dyck CH, Rosenblatt W, Zea-Ponce Y, Zoghbi SS, Baldwin RM, Charney DS, Hoffer PB, Kung HF, Innis RB: SPECT imaging of striatal dopamine release after amphetamine challenge. J Nucl Med 1995; 36:1182–1190Google Scholar
17. Talairach J, Tournoux P: Co-Planar Stereotaxic Atlas of the Human Brain: Three-Dimensional Proportional System. New York, Thieme Medical, 1988Google Scholar
18. Volkow ND, Fowler JS, Wang GJ, Hitzemann R, Logan J, Schlyer DJ, Dewey SL, Wolf AP: Decreased dopamine D2 receptor availability is associated with reduced frontal metabolism in cocaine abusers. Synapse 1993; 14:169–177Google Scholar
19. Drevets WC, Gautier C, Price JC, Kupfer DJ, Kinahan PE, Grace AA, Price JL, Mathis CA: Amphetamine-induced dopamine release in human ventral striatum correlates with euphoria. Biol Psychiatry 2001; 49:81–96Google Scholar
20. Kalivas PW, Duffy P: Time course of extracellular dopamine and behavioral sensitization to cocaine, I: dopamine axon terminals. J Neurosci 1993; 13:276–284Google Scholar
21. Pettit HO, Pan HT, Parsons LH, Justice JBJ: Extracellular concentration of cocaine and dopamine are enhanced during chronic cocaine administration. J Neurochem 1990; 55:798–804Google Scholar
22. Bradberry CW: Acute and chronic dopamine dynamics in a nonhuman primate model of recreational cocaine use. J Neurosci 2000; 20:7109–7115Google Scholar
23. Beitner-Johnson D, Guitart X, Nestler EJ: Neurofilament proteins and the mesolimbic dopamine system: common regulation by chronic morphine and chronic cocaine in the rat ventral tegmental area. J Neurosci 1992; 12:2165–2176Google Scholar
24. Robertson MW, Leslie CA, Bennett JP Jr: Apparent synaptic dopamine deficiency induced by withdrawal from chronic cocaine treatment. Brain Res 1991; 538:337–339Google Scholar
25. Rossetti ZL, Hmaidan Y, Gessa GL: Marked inhibition of mesolimbic dopamine release: a common feature of ethanol, morphine, cocaine and amphetamine abstinence in rats. Eur J Pharmacol 1992; 221(2–3):227–234Google Scholar
26. Mateo Y, Lack CM, Morgan D, Roberts DC, Jones SR: Reduced dopamine terminal function and insensitivity to cocaine following cocaine binge self-administration and deprivation. Neuropsychopharmacology 2005; 30:1455–1463Google Scholar
27. Little KY, Zhang L, Desmond T, Frey KA, Dalack GW, Cassin BJ: Striatal dopaminergic abnormalities in human cocaine users. Am J Psychiatry 1999; 156:238–245Google Scholar
28. Little KY, Krolewski DM, Zhang L, Cassin BJ: Loss of striatal vesicular monoamine transporter protein (VMAT2) in human cocaine users. Am J Psychiatry 2003; 160:47–55Google Scholar
29. Wu JC, Bell K, Najafi A, Widmark C, Keator D, Tang C, Klein E, Bunney BG, Fallon J, Bunney WE: Decreasing striatal 6-FDOPA uptake with increasing duration of cocaine withdrawal. Neuropsychopharmacology 1997; 17:402–409Google Scholar
30. Laruelle M, Iyer RN, Al-Tikriti MS, Zea-Ponce Y, Malison R, Zoghbi SS, Baldwin RM, Kung HF, Charney DS, Hoffer PB, Innis RB, Bradberry CW: Microdialysis and SPECT measurements of amphetamine-induced dopamine release in nonhuman primates. Synapse 1997; 25:1–14Google Scholar
31. Breier A, Su TP, Saunders R, Carson RE, Kolachana BS, deBartolomeis A, Weinberger DR, Weisenfeld N, Malhotra AK, Eckelman WC, Pickar D: Schizophrenia is associated with elevated amphetamine-induced synaptic dopamine concentrations: evidence from a novel positron emission tomography method. Proc Natl Acad Sci USA 1997; 94:2569–2574Google Scholar
32. Wise RA: Neurobiology of addiction. Curr Opin Neurobiol 1996; 6:243–251Google Scholar
33. Hassani OK, Cromwell HC, Schultz W: Influence of expectation of different rewards on behavior-related neuronal activity in the striatum. J Neurophysiol 2001; 85:2477–2489Google Scholar
34. Berridge KC, Robinson TE: What is the role of dopamine in reward: hedonic impact, reward learning, or incentive salience? Brain Res Brain Res Rev 1998; 28:309–369Google Scholar
35. Salamone JD, Correa M, Mingote S, Weber SM: Nucleus accumbens dopamine and the regulation of effort in food-seeking behavior: implications for studies of natural motivation, psychiatry, and drug abuse. J Pharmacol Exp Ther 2003; 305:1–8Google Scholar
36. Bindra D: A motivational view of learning, performance, and behavior modification. Psychol Rev 1974; 81:199–213Google Scholar