Lower Expression of Glutamic Acid Decarboxylase 67 in the Prefrontal Cortex in Schizophrenia: Contribution of Altered Regulation by Zif268
Abstract
Objective
Cognitive deficits of schizophrenia may be due at least in part to lower expression of the 67-kDa isoform of glutamic acid decarboxylase (GAD67), a key enzyme for GABA synthesis, in the dorsolateral prefrontal cortex of individuals with schizophrenia. However, little is known about the molecular regulation of lower cortical GAD67 levels in schizophrenia. The GAD67 promoter region contains a conserved Zif268 binding site, and Zif268 activation is accompanied by increased GAD67 expression. Thus, altered expression of the immediate early gene Zif268 may contribute to lower levels of GAD67 mRNA in the dorsolateral prefrontal cortex in schizophrenia.
Method
The authors used polymerase chain reaction to quantify GAD67 and Zif268 mRNA levels in dorsolateral prefrontal cortex area 9 from 62 matched pairs of schizophrenia and healthy comparison subjects, and in situ hybridization to assess Zif268 expression at laminar and cellular levels of resolution. The effects of potentially confounding variables were assessed in human subjects, and the effects of antipsychotic treatments were tested in antipsychotic-exposed monkeys. The specificity of the Zif268 findings was assessed by quantifying mRNA levels for other immediate early genes.
Results
GAD67 and Zif268 mRNA levels were significantly lower and were positively correlated in the schizophrenia subjects. Both Zif268 mRNA-positive neuron density and Zif268 mRNA levels per neuron were significantly lower in the schizophrenia subjects. These findings were robust to the effects of the confounding variables examined and differed from other immediate early genes.
Conclusions
Deficient Zif268 mRNA expression may contribute to lower cortical GAD67 levels in schizophrenia, suggesting a potential mechanistic basis for altered cortical GABA synthesis and impaired cognition in schizophrenia.
Impaired working memory performance, a core component of cognitive dysfunction in schizophrenia, is associated with altered activity of the dorsolateral prefrontal cortex. The alterations are thought to be due at least in part to disturbances in GABA neurotransmission (1). For example, mRNA (2–8) and protein (9, 10) levels of the 67-kDa isoform of glutamic acid decarboxylase (GAD67), a key enzyme for cortical GABA synthesis, have been consistently reported to be lower in the dorsolateral prefrontal cortex of subjects with schizophrenia. The frequency with which lower cortical GAD67 levels has been observed in schizophrenia suggests that it is a conserved feature of the disease process. In addition, other observations suggest that lower cortical GAD67 levels are not a consequence of illness chronicity (11) or of other factors that frequently accompany schizophrenia (9). Since GAD67 expression is heavily regulated by neuronal or network activity (12, 13), disease-related alterations in activity-dependent regulatory factors may contribute to lower GAD67 levels (14).
One activity-dependent regulatory factor that may regulate GAD67 expression is the immediate early gene Zif268 (also termed EGR-1, NGFI-A, and Krox-24), which is rapidly and transiently expressed in response to neuronal activation. The GAD67 promoter region contains a conserved Zif268 binding site (15, 16), and Zif268 activation is accompanied by increased GAD67 expression in rat hippocampal neurons (17). In contrast to other immediate early genes, Zif268 is expressed at stable, relatively high basal levels in the brain, and the expression of Zif268 is layer-specific in the cortex (18–23). Together, these data suggest that Zif268 mRNA expression in certain neuronal populations may play an important role in maintaining baseline cortical physiology while also regulating gene expression in response to particular stimuli. Given reports of lower levels of Zif268 mRNA in the dorsolateral prefrontal cortex from small samples of subjects with schizophrenia (24, 25), reduced cortical Zif268 expression in schizophrenia may be responsible for lower GAD67 mRNA levels in the illness. However, neither the relation between Zif268 and GAD67 expression nor the molecular and cellular specificity of altered Zif268 mRNA expression has been directly examined in schizophrenia. For example, since immediate early genes are generally expressed in an activity-dependent manner (26), it is important to determine whether changes in Zif268 mRNA expression differ from those of other immediate early genes in the dorsolateral prefrontal cortex of subjects with schizophrenia.
To address these questions, we used quantitative polymerase chain reaction (qPCR) and in situ hybridization to determine expression levels of Zif268 mRNA in the dorsolateral prefrontal cortex from a large cohort of schizophrenia and matched nonpsychiatric comparison subjects, the within-subject relationship between Zif268 and GAD67 mRNA levels, and the effects of potentially confounding variables. To determine the specificity of the relationship between Zif268 and GAD67 mRNA levels, we also quantified the expression of other regulatory immediate early genes (c-fos, c-jun, and EGR-2).
Method
Human Subjects
Brain specimens (N=124) were obtained during autopsies conducted at the Allegheny County Office of the Medical Examiner (Pittsburgh) after consent was obtained from the next of kin. An independent committee of experienced research clinicians made consensus DSM-IV diagnoses for each subject, based on the results of structured interviews with family members and review of medical records; the same approach was used to confirm the absence of psychiatric diagnoses in comparison subjects (27). Tissue was collected from dorsolateral prefrontal cortex area 9, and samples were prepared as described previously (7, 27) (see the data supplement that accompanies the online edition of this article). All procedures were approved by the University of Pittsburgh’s Committee for the Oversight of Research and Clinical Trials Involving the Dead and the Institutional Review Board for Biomedical Research.
To control for experimental variance and to reduce biological variance between groups, each subject with schizophrenia or schizoaffective disorder (N=62) was matched with one nonpsychiatric comparison subject for sex and as closely as possible for age (Table 1). The groups did not differ significantly in mean age, postmortem interval, RNA integrity number (Agilent Bioanalyzer, Santa Clara, Calif.), or tissue storage time at −80°C (see Table S1 in the data supplement for demographic details on each subject). Although brain pH significantly differed between groups (t=2.68; df=61, p=0.01), the mean difference was very small (0.1 pH unit) and of uncertain biological significance. Each subject had an RNA integrity number ≥7.0, indicating an excellent quality of total RNA.
Characteristic | Comparison Group (N=62) | Schizophrenia Group (N=62) | ||
---|---|---|---|---|
N | % | N | % | |
Male | 47 | 76 | 47 | 76 |
White | 52 | 84 | 46 | 74 |
Black | 10 | 16 | 16 | 26 |
Mean | SD | Mean | SD | |
Age (years) | 48.7 | 13.8 | 47.7 | 12.7 |
Postmortem interval (hours) | 18.8 | 5.5 | 19.2 | 8.5 |
Brain pH | 6.7 | 0.2 | 6.6 | 0.3 |
RNA integrity number | 8.2 | 0.6 | 8.1 | 0.6 |
Storage time (months at −80°C) | 123.2 | 56.9 | 119.4 | 61.4 |
qPCR
Total RNA (10 ng/μL) was converted into complementary DNA (cDNA) for each subject using a High-Capacity cDNA Reverse Transcription Kit (Life Technologies, Carlsbad, Calif.). The cycle threshold was determined for each transcript of interest using Power SYBR Green Master Mix (Life Technologies) and the ViiA 7 Real-Time PCR System (Life Technologies) using ViiA 7 software according to the manufacturer’s instructions. cDNA samples from both subjects in a pair were processed together in quadruplicate on the same 384-well plate. Based on their stable levels of expression across schizophrenia and comparison subjects (28), three internal reference transcripts (β-actin, cyclophilin A, and glyceraldehyde-3-phosphate dehydrogenase) were used to normalize the target transcripts. All primer sets in this study showed ≥96% amplification efficiency in individual standard curve analyses and amplified a specific single product in dissociation curve analyses (see Table S2A in the online data supplement). The relative expression level of each target transcript was determined using the 2−Δcycle threshold method as described previously (28) (see the online data supplement).
In Situ Hybridization
Templates for the synthesis of riboprobes against human Zif268 were first generated by PCR. The specific primer set amplified a 427-pair fragment corresponding to bases 1461–1887 of the human Zif268 gene (GenBank NM_001964), which was nonoverlapping with the primer set used in the qPCR study. Nucleotide sequencing confirmed 100% homology of the amplified fragment to the reported sequence (see Table S2B in the online data supplement). Sense and antisense riboprobes were transcribed in vitro in the presence of 35S-CTP (PerkinElmer, Waltham, Mass.), and in situ hybridization procedures were performed with three tissue sections from each subject as described previously (27, 29) (see the online data supplement). The hybridized sections were exposed to autoradiographic films, coated with nuclear emulsion, developed, and counterstained with cresyl violet.
Zif268 mRNA levels in the total gray matter and individual cortical layers of area 9 were measured as film optical densities (27, 29) (see the online data supplement). To evaluate Zif268 mRNA expression at the cellular level, the numbers of silver grains generated by the 35S-labeled riboprobe in emulsion-coated sections were counted in sampling frames placed in layers deep 3–4 (see the online data supplement, and Figure S1). Within the sampling frame, grain numbers were counted within 22-μm diameter circles placed over each Nissl-stained nucleus, previously determined to cover the largest cross-sectional area of interneurons in human dorsolateral prefrontal cortex (7, 30). Neurons were considered to be specifically labeled, referred to as Zif268-positive neurons, if the grain number per neuron was more than five times the background density, as determined for each section by counting the grains in the same circle placed over glial nuclei (27, 29).
Antipsychotic-Exposed Monkeys
Young adult male monkeys (Macaca fasicularis) received twice-daily oral doses of haloperidol, olanzapine, or placebo (N=6 monkeys per group) for 17–27 months, as described previously (31). The final trough plasma levels for haloperidol and olanzapine were within the range associated with clinical efficacy in humans. Monkeys were matched by terminal body weight and euthanized in triads (one animal from each group) on the same day. Coronal sections (16 μm) from the right frontal lobe were cut from fresh-frozen tissue blocks containing the middle one-third of the principal sulcus. Two serial sections from each animal, evenly spaced at 224 μm, were used for in situ hybridization to measure Zif268 mRNA expression in areas 9 and 46 as described above. All studies were carried out in accordance with the National Institutes of Health Guide for the Care and Use of Laboratory Animals and were approved by the University of Pittsburgh.
Statistical Analysis
Using two analysis of covariance (ANCOVA) models, paired and unpaired, we tested the effects of diagnostic group on gene expression levels determined by qPCR and for total, laminar, and cellular expression levels of Zif268 mRNA determined by in situ hybridization. The paired ANCOVA model used diagnostic group as the main effect, subject pair as a blocking factor, and postmortem interval, storage time, brain pH, and RNA integrity number as covariates. Subject pairing may be considered an attempt to balance diagnostic groups for sex and age and to account for the parallel processing of tissue samples from a pair, and thus is not a true statistical paired design. Therefore, in order to validate the paired model, the unpaired ANCOVA model was applied with diagnostic group as main effect and sex, age, postmortem interval, storage time, brain pH, and RNA integrity number as covariates. Within each subject group, subjects with transcript levels that were more than three standard deviations from the group mean were considered outliers for that measure and removed from subsequent analyses. For this reason, the degrees of freedom differ slightly across analyses.
The potential influence of other factors (e.g., diagnosis of schizoaffective disorder; history of substance dependence or abuse; nicotine use at time of death; antipsychotic, antidepressant, benzodiazepine, or sodium valproate use at time of death; or death by suicide) on Zif268 mRNA expression levels in the schizophrenia group were assessed by using an ANCOVA model with each factor as the main effect and sex, age, postmortem interval, storage time, brain pH, and RNA integrity number as covariates. The relationship between each immediate early gene and GAD67 mRNA levels was assessed by Pearson’s correlation. For the antipsychotic-exposed monkeys, ANCOVA models were used with treatment group as a main effect and triad as a blocking factor. All p values were two-tailed, and the significance threshold was set at 0.05.
Results
Zif268 mRNA Expression in Schizophrenia
By qPCR, the mean level of Zif268 mRNA was significantly 32% lower in schizophrenia subjects (paired: F=21.5, df=1, 53, p<0.001; unpaired: F=29.6, df=1, 111, p<0.001). Relative to their matched comparison subjects, 49 of the 62 schizophrenia subjects had lower Zif268 mRNA expression (Figure 1A). In the unpaired ANCOVA model, age was a significant determinant of Zif268 mRNA expression in the gray matter (F=19.0, df=1, 111, p<0.001). Further analyses demonstrated that Zif268 mRNA levels were similarly negatively correlated with age in schizophrenia subjects (N=58; r=−0.38, p=0.003) and comparison subjects (N=61; r=−0.39, p=0.002) (Figure 1B).

a qPCR=quantitative polymerase chain reaction. In panel A, the scatterplots indicate Zif268 mRNA level for each comparison and schizophrenia subject in a pair. Data points below the diagonal unity line indicate lower levels for Zif268 mRNA in the schizophrenia subject relative to the comparison subject. Mean Zif268 mRNA level was significantly lower in the schizophrenia subjects relative to the comparison subjects (mean=0.041 [SD=0.023] and mean=0.059 [SD=0.020], respectively; −32%; F=21.5, df=1, 53, p<0.001). In panel B, Zif268 mRNA levels in dorsolateral prefrontal cortex were significantly negatively correlated with age in both subject groups (schizophrenia subjects: N=58; r=−0.38, p=0.003; comparison subjects: N=61; r=−0.39, p=0.002). In panel C, in which schizophrenia subjects are grouped by potential confounding factors, the circles represent Zif268 mRNA expression levels for individual subjects and the bars represent mean Zif268 mRNA levels for the indicated group. Schizophrenia subjects taking benzodiazepines or sodium valproate at the time of death had lower Zif268 mRNA levels relative to those not taking these medications (F=7.64, df=1, 50, p=0.008).
b Information on nicotine use was not available for all subjects.
c Cause of death was undetermined for one schizophrenia subject.
Levels of Zif268 mRNA in schizophrenia subjects did not differ as a function of sex, diagnosis of schizoaffective disorder, history of substance dependence or abuse, nicotine use at the time of death, use of antidepressants or antipsychotics at the time of death, or death by suicide (Figure 1C). Schizophrenia subjects taking benzodiazepines or sodium valproate at the time of death had significantly lower Zif268 mRNA levels relative to those not taking these medications (F=7.64, df=1, 50, p=0.008) (Figure 1C). However, even those who were not taking these medications at the time of death had significantly lower Zif268 mRNA expression compared with their matched comparison subjects (−16.7%; paired: F=4.21, df=1, 29, p=0.049; unpaired: F=4.13, df=1, 60, p=0.047). In addition, to evaluate the potential effect of long-term exposure to conventional and atypical antipsychotics, we used in situ hybridization to measure the levels of Zif268 mRNA in the dorsolateral prefrontal cortex of monkeys chronically exposed to haloperidol, olanzapine, or placebo. Levels of Zif268 mRNA did not differ among these three groups of monkeys (see Figure S2 in the online data supplement).
Localization of Lower Zif268 mRNA in Schizophrenia
To examine the nature of lower Zif268 mRNA levels in schizophrenia at the laminar and cellular levels, we used in situ hybridization to measure Zif268 mRNA levels in the dorsolateral prefrontal cortex from 41 subject pairs with a sufficient number of available tissue sections (see Table S1 in the online data supplement). Mean tissue levels of Zif268 mRNA in the schizophrenia subjects were similarly lower when quantified by in situ hybridization (−34.0%; paired: F=15.4, df=1, 34, p<0.001; unpaired: F=23.6, df=1, 71, p<0.001) (Figure 2A,B) or by qPCR (−29.3%). Furthermore, levels of Zif268 mRNA quantified by qPCR or in situ hybridization were highly correlated (N=78; r=0.76, p<0.001) within subjects. Given that the PCR primer set and in situ hybridization probe were directed at nonoverlapping sequences of Zif268 mRNA, these findings robustly support a deficit in Zif268 expression in schizophrenia.
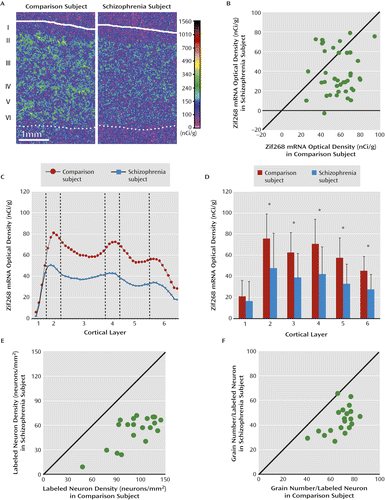
a Panel A presents representative pseudocolored film autoradiographs of dorsolateral prefrontal cortex sections illustrating Zif268 mRNA expression levels in a schizophrenia and matched comparison subject pair. The solid and dotted lines indicate the pial surface and the gray/white matter border, respectively. In panel B, the scatterplot indicates average Zif268 mRNA levels across the gray matter for each comparison and schizophrenia subject pair. Data points below the diagonal unity line indicate lower Zif268 mRNA levels in the schizophrenia subject relative to the matched comparison subject. Mean Zif268 mRNA levels were significantly lower in schizophrenia subjects relative to comparison subjects (mean=38.0 [SD=22.1] and mean=57.6 [SD=15.4], respectively; −34.0%; F=15.4, df=1, 34, p<0.001). Panels C and D show laminar expression of mean Zif268 mRNA levels across all cortical layers (panel C) and in each cortical layer (panel D) in schizophrenia and comparison subjects (error bars indicate standard deviation). Laminar analysis revealed that mean Zif268 mRNA levels were lower by 36.8% in layer 2, 37.7% in layer 3, 40.6% in layer 4, 42.8% in layer 5, and 38.7% in layer 6 in schizophrenia subjects relative to comparison subjects. Panels E and F present scatterplots of Zif268-positive neurons per mm2 and grains per positive neuron, respectively, in layers deep 3–4 for each comparison and schizophrenia subject pair. Data points below the diagonal unity lines indicate lower Zif268 mRNA levels in the schizophrenia subject relative to the comparison subject. Cellular analysis revealed that the mean number of Zif268-positive neurons/mm2 was lower in layers deep 3–4 in schizophrenia subjects relative to comparison subjects (mean=53.8 [SD=18.3] and mean=112.7 [ SD=24.4], respectively; −52.3%; F=266.3, df=1, 15, p<0.001). The mean grain density per Zif268-positive neuron was also lower in layers deep 3–4 in schizophrenia subjects relative to comparison subjects (mean=43.8 [SD=10.8] and mean=68.1 [SD=10.5], respectively; −35.6%; F=71.4, df=1, 15, p<0.001).
*p<0.001.
Analysis by cortical layers revealed that Zif268 mRNA expression was significantly lower in layers 2–6 (paired: all F values >15.2 (df=1, 34), all p values <0.001; unpaired: all F values >23.1 (df=1, 71), all p values <0.001), but not in layer 1 (Figure 2C,D). Across layers 2–6, the largest percentage difference in the schizophrenia subjects was present in layer 5 (−42.8%) and the smallest difference in layer 2 (−36.8%).
To determine whether all or a subset of Zif268-positive cortical neurons were affected, we performed grain counting analysis in layers deep 3–4 in a subset of 20 subject pairs in which the total cortical expression level was lower by ≥40% in the schizophrenia subject relative to the matched comparison subject (see Figure S1 in the online data supplement). In the schizophrenia subjects, the mean number of Zif268-positive neurons per mm2 was lower (−52.3%; paired: F=266.3, df=1, 15, p<0.001; unpaired: F=68.0, df=1, 32, p<0.001) and the mean grain density per Zif268-positive neuron was lower (−35.6%; paired: F=71.4, df=1, 15, p<0.001; unpaired: F=36.9, df=1, 32, p<0.001) relative to comparison subjects (Figure 2E,F). The magnitude of these reductions in Zif268 mRNA levels did not differ between the 11 schizophrenia subjects who were taking benzodiazepines or sodium valproate at the time of death and nine subjects who were not (see Table S1 in the online data supplement). These data suggest that in schizophrenia a subset of neurons fail to express detectable levels of Zif268 mRNA and other neurons express lower Zif268 mRNA levels.
Correlation of Zif268 and GAD67 mRNA Expression
To examine the relationship between the changes in Zif268 and GAD67 expression in the dorsolateral prefrontal cortex, we used GAD67 mRNA levels previously reported in the first 42 subject pairs of our cohort (9) and quantified GAD67 mRNA in the remaining 20 pairs by qPCR (see Table S1 in the online data supplement). GAD67 mRNA levels were significantly lower in the schizophrenia subjects from the new 20-pair cohort (−18.3%; paired: F=4.81, df=1, 15, p=0.044; unpaired: F=6.32, df=1, 32, p=0.017) and in the combined 62-pair cohort (−14.3%; paired: F=12.6, df=1, 57, p=0.001; unpaired: F=12.8, df=1, 116, p=0.001). Relative to their matched comparison subjects, 45 of the 62 schizophrenia subjects had lower GAD67 mRNA levels (Figure 3A). Levels of Zif268 and GAD67 mRNA as determined by qPCR were positively correlated in schizophrenia subjects (N=58; r=0.29, p=0.027) but not in comparison subjects (N=61; r=−0.15, p=0.25) (Figure 3B). In the schizophrenia subjects who were not taking benzodiazepines or sodium valproate at the time of death, Zif268 mRNA levels were also significantly correlated with GAD67 mRNA levels (N=34; r=0.37, p=0.030). These data support the idea that lower expression of Zif268 mRNA may be causally related to lower expression of GAD67 mRNA in schizophrenia.
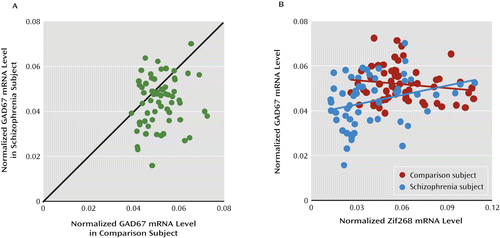
a qPCR=quantitative polymerase chain reaction. In panel A, the scatterplot indicates GAD67 mRNA level for each schizophrenia and matched comparison subject pair. Data points below the diagonal unity line indicate lower levels for GAD67 mRNA in the schizophrenia subject relative to the matched comparison subject. Mean mRNA levels for GAD67 were significantly lower in schizophrenia subjects relative to comparison subjects (mean=0.044 [SD=0.011] and mean=0.052 [SD=0.008], respectively; −14.3%; F=12.6, df=1, 57, p=0.001). In panel B, Zif268 mRNA levels were positively correlated with GAD67 mRNA levels in schizophrenia subjects (N=58; r=0.29, p=0.027), but not in comparison subjects (N=61; r=−0.15, p=0.25).
Expression of Other Immediate Early Gene mRNA in Schizophrenia
To determine the specificity of the lower Zif268 mRNA levels in the dorsolateral prefrontal cortex of schizophrenia subjects, we used qPCR to quantify mRNA levels of three other immediate early genes (c-fos, c-jun and EGR-2) in the 62 subject pairs (Figure 4). These three genes are the prototypical members of the fos/jun and EGR families and, like Zif268, are known to be regulatory immediate early genes. Mean levels of c-fos mRNA did not differ between subject groups, mean levels of c-jun mRNA were significantly higher in schizophrenia subjects (+34.2%; paired: F=10.9, df=1, 56, p=0.002; unpaired: F=14.8, df=1, 115, p<0.001), and mean levels of EGR-2 mRNA were lower in schizophrenia subjects (−23.8%), but the difference fell short of significance (paired: F=3.49, df=1, 55, p=0.067; unpaired: F=3.81, df=1, 114, p=0.053). None of the mRNA levels for these immediate early genes were significantly correlated with GAD67 mRNA levels in either schizophrenia or comparison subjects. These data demonstrate that mRNA levels of regulatory immediate early genes are not uniformly lower in the dorsolateral prefrontal cortex of schizophrenia subjects.
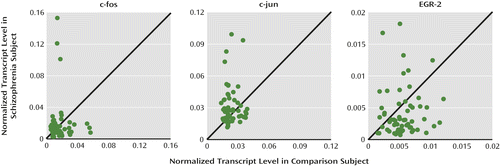
a qPCR=quantitative polymerase chain reaction. Scatterplots indicate mRNA levels of immediate early genes for each schizophrenia and matched comparison subject pair. Data points below the diagonal unity lines indicate lower mRNA levels in the schizophrenia subject relative to the comparison subject and vice versa. Mean mRNA level for c-fos did not differ between schizophrenia (mean=0.018, SD=0.026) and comparison subjects (mean=0.017, SD=0.012). In contrast, mean mRNA levels for c-jun were statistically higher in schizophrenia subjects relative to comparison subjects (mean=0.031 [SD=0.018] and mean=0.023 [SD=0.007], respectively; +34.2%; F=10.9, df=1, 56, p=0.002), while mean mRNA level for EGR-2 was lower, but fell short of significance, in schizophrenia subjects relative to comparison subjects (mean=0.0042 [SD=0.0038] and mean=0.0056 [SD=0.0024], respectively; −23.8%; F=3.49, df=1, 55, p=0.067).
Discussion
Lower levels of GAD67 mRNA and protein have been consistently found in the dorsolateral prefrontal cortex of subjects with schizophrenia (2–10). In this study, we focused on whether altered expression of the transcriptional regulatory factor Zif268 might contribute to lower cortical GAD67 levels in schizophrenia subjects. Consistent with the results of previous studies in small cohorts (24, 25), we found that Zif268 mRNA levels were significantly lower in the dorsolateral prefrontal cortex of schizophrenia subjects. Furthermore, we replicated previous findings of lower GAD67 mRNA levels (9) in a new cohort of schizophrenia subjects. Because Zif268 binds to the promoter region of the GAD1 gene and can regulate GAD67 expression (17), we hypothesized that deficient expression of Zif268 might be “upstream” of lower GAD67 mRNA expression in the dorsolateral prefrontal cortex of schizophrenia subjects. Consistent with this hypothesis, the expression levels of Zif268 and GAD67 mRNAs were positively correlated in the schizophrenia subjects. In addition, mRNA levels of other representative regulatory immediate early genes were not correlated with GAD67 mRNA levels in either schizophrenia or comparison subjects, suggesting that lower expression of Zif268 mRNA might be specific in schizophrenia, and thus contribute to lower cortical GAD67 levels in the disorder. However, the present study cannot exclude the possibility that lower GAD67 mRNA expression in schizophrenia is also regulated by other mechanisms. For example, allelic variants in the GAD1 gene that are associated with risk for schizophrenia (32) and altered histone methylation of the promoter regions of GAD1 (33) both appear to affect GAD67 mRNA levels in the dorsolateral prefrontal cortex, suggesting that multiple factors may contribute to lower cortical GAD67 levels in schizophrenia. Alternatively, since not all schizophrenia subjects showed lower Zif268 or GAD67 expression, lower levels of Zif268 mRNA may drive altered GAD67 expression in only a subset of individuals with schizophrenia (27).
Our in situ hybridization study revealed a distinctive laminar pattern of Zif268 expression in human dorsolateral prefrontal cortex similar to that reported for the rodent, cat, and monkey cortex (18, 19, 21–23). Specifically, Zif268 mRNA-positive neurons were distributed across layers 2–6, with the highest densities in layers 2 and 4. Consistent with the qPCR findings, Zif268 mRNA levels were significantly lower in layers 2–6 in schizophrenia subjects relative to comparison subjects. At the cellular level, we found that a subset of neurons failed to express detectable levels of Zif268 mRNA and that other neurons expressed lower Zif268 mRNA levels in the dorsolateral prefrontal cortex of schizophrenia subjects. Together, the lower levels of Zif268 mRNA, which were demonstrated at different levels of resolution using two different methods, support the interpretation that Zif268 mRNA levels are lower in the dorsolateral prefrontal cortex of schizophrenia subjects and thus may contribute to lower expression of GAD67.
Although other classes of GABA neurons may be affected, only the subset of GABA neurons containing the calcium-binding protein parvalbumin have been directly shown to have lower GAD67 mRNA expression in schizophrenia (30). Recently, we successfully performed microarray analyses from individually dissected neurons labeled with Vicia villosa agglutinin, which is present in the perineuronal nets that surround parvalbumin neurons (29). In the healthy comparison subjects used in the present study, Zif268 mRNA was highly expressed in the labeled neurons. This level of expression was similar to that of parvalbumin and was much higher than that of any other regulatory immediate early genes. In the labeled neurons, the mean level of Zif268 mRNA was 16% lower in the schizophrenia subjects relative to the comparison subjects, although this difference did not achieve statistical significance. The smaller magnitude of this difference relative to that observed by in situ hybridization may reflect the fact that only parvalbumin neurons with relatively well preserved perineuronal nets were sampled in the schizophrenia subjects; that is, the density of perineuronal nets identified by Vicia villosa agglutinin labeling is lower in schizophrenia (34, 35), suggesting that the most severely affected parvalbumin neurons were not captured by laser microdissection (29). Furthermore, for the 14 subject pairs used in the microarray study, our qPCR study of gray matter found that Zif268 mRNA levels were only 22% lower in the schizophrenia subjects, compared with the 34% deficit observed in the remaining 48 schizophrenia subjects of the entire cohort of 62 subject pairs. These data indicate that Zif268 mRNA is heavily expressed in the parvalbumin population of GABA neurons and suggest that lower Zif268 mRNA expression in those neurons may contribute to lower GAD67 mRNA levels in schizophrenia.
Several lines of evidence indicate that the lower Zif268 mRNA expression is due to the disease process of schizophrenia and is not attributable to potential confounding factors frequently associated with the illness. First, none of the potentially confounding clinical factors, such as sex, diagnosis of schizoaffective disorder, history of substance dependence or abuse, nicotine use at the time of death, use of antipsychotics or antidepressants at the time of death, or death by suicide, accounted for lower Zif268 mRNA expression in the schizophrenia subjects. Although the use of benzodiazepines or sodium valproate at the time of death was associated with lower Zif268 mRNA expression, schizophrenia subjects who had not taken these medications still had significantly lower Zif268 mRNA levels than their matched comparison subjects. Second, Zif268 mRNA expression did not differ in the dorsolateral prefrontal cortex of monkeys chronically exposed to either haloperidol or olanzapine. Finally, a significant negative correlation between age and Zif268 mRNA level was seen in both schizophrenia and comparison subjects. The regression line for schizophrenia subjects was parallel to and shifted downward from that of comparison subjects, indicating that lower expression of Zif268 mRNA in schizophrenia subjects is present across adult life and thus is unlikely to be a consequence of illness chronicity. However, we cannot exclude the possibility that Zif268 mRNA expression declines during the early stages of clinical illness after onset of psychosis. Furthermore, this observation suggests that the deficient expression of Zif268 mRNA might be present early in the course of the illness and thus could contribute to the pathophysiology of the clinical features of the illness that reflect altered GABA neurotransmission.
Since all immediate early genes are activity dependent, it is possible that lower Zif268 mRNA levels in schizophrenia simply reflect a nonspecific consequence of reduced cortical neuronal activity associated with prefrontal cortex dysfunction (“hypofrontality”). However, this interpretation seems unlikely since 1) lower Zif268 mRNA levels were not related to illness chronicity or other factors that frequently accompany schizophrenia and 2) mRNA levels of other regulatory immediate early genes examined in the present study were not lower in the schizophrenia subjects. In fact, the other three immediate early genes examined were lower, unchanged, or higher in expression in schizophrenia subjects. Consistent with these results, accumulating evidence suggests that the expression of each immediate early gene can be individually regulated by selective signaling pathways. For example, various stimulation paradigms that induce long-term potentiation result in increased mRNA levels of Zif268 but not of other members of the fos/jun family (36, 37). Likewise, high-frequency electrical stimulation of the mediodorsal thalamic nucleus was found to result in increased expression of Zif268 mRNA, but not of c-fos mRNA, in rat frontal cortex (38). Taken together, these findings suggest that the Zif268 signaling pathway may be selectively altered in schizophrenia, and thus contribute to lower cortical GAD67 levels in the disorder.
Of course, the factors leading to lower Zif268 mRNA expression in a majority of schizophrenia subjects remain to be determined. One possibility could be reduced activity in specific circuits of the dorsolateral prefrontal cortex, such as those furnished by layer 3 pyramidal cells (14). These cells have a lower density of dendritic spines (39, 40), the principal site of excitatory synaptic inputs, and thus they presumably receive less excitatory drive from other cortical regions or the thalamus (41). The axons of dorsolateral prefrontal cortex layer 3 pyramidal cells project across all cortical layers (42), and thus reduced activity in these cells could account for the observed laminar distribution of lower Zif268 mRNA expression in subjects with schizophrenia.
In summary, the findings of this study suggest that lower levels of Zif268 mRNA may be one upstream molecular factor leading to lower GAD67 mRNA levels in schizophrenia, providing a potential mechanistic basis for impaired GABA synthesis in the illness.
1 : Cortical inhibitory neurons and schizophrenia. Nat Rev Neurosci 2005; 6:312–324Crossref, Medline, Google Scholar
2 : Gene expression for glutamic acid decarboxylase is reduced without loss of neurons in prefrontal cortex of schizophrenics. Arch Gen Psychiatry 1995; 52:258–266Crossref, Medline, Google Scholar
3 : Prefrontal GABA(A) receptor alpha-subunit expression in normal postnatal human development and schizophrenia. J Psychiatr Res 2010; 44:673–681Crossref, Medline, Google Scholar
4 : Relationship of brain-derived neurotrophic factor and its receptor TrkB to altered inhibitory prefrontal circuitry in schizophrenia. J Neurosci 2005; 25:372–383Crossref, Medline, Google Scholar
5 : Decreased glutamic acid decarboxylase(67) mRNA expression in multiple brain areas of patients with schizophrenia and mood disorders. J Psychiatr Res 2009; 43:970–977Crossref, Medline, Google Scholar
6 : Microarray analysis of gene expression in the prefrontal cortex in schizophrenia: a preliminary study. Schizophr Res 2002; 58:11–20Crossref, Medline, Google Scholar
7 : Decreased glutamic acid decarboxylase67 messenger RNA expression in a subset of prefrontal cortical gamma-aminobutyric acid neurons in subjects with schizophrenia. Arch Gen Psychiatry 2000; 57:237–245Crossref, Medline, Google Scholar
8 : Disease-specific alterations in glutamatergic neurotransmission on inhibitory interneurons in the prefrontal cortex in schizophrenia. Brain Res 2008; 1218:267–277Crossref, Medline, Google Scholar
9 : Cortical deficits of glutamic acid decarboxylase 67 expression in schizophrenia: clinical, protein, and cell type-specific features. Am J Psychiatry 2011; 168:921–929Link, Google Scholar
10 : Decrease in reelin and glutamic acid decarboxylase67 (GAD67) expression in schizophrenia and bipolar disorder: a postmortem brain study. Arch Gen Psychiatry 2000; 57:1061–1069Crossref, Medline, Google Scholar
11 : Altered cortical expression of GABA-related genes in schizophrenia: illness progression vs developmental disturbance. Schizophr Bull (Epub ahead of print, Dec 22, 2013)Google Scholar
12 : Activity-dependent changes in GAD and preprotachykinin mRNAs in visual cortex of adult monkeys. Cereb Cortex 1994; 4:40–51Crossref, Medline, Google Scholar
13 : Activity-dependent regulation of inhibition via GAD67. J Neurosci 2012; 32:8521–8531Crossref, Medline, Google Scholar
14 : Cortical parvalbumin interneurons and cognitive dysfunction in schizophrenia. Trends Neurosci 2012; 35:57–67Crossref, Medline, Google Scholar
15 : Structure and the promoter region of the mouse gene encoding the 67-kD form of glutamic acid decarboxylase. DNA Cell Biol 1996; 15:1081–1091Crossref, Medline, Google Scholar
16 : Structure and alternative promoters of the mouse glutamic acid decarboxylase 67 gene. Biochem J 1997; 326:573–578Crossref, Medline, Google Scholar
17 : SDF1alpha/CXCR4 signaling, via ERKs and the transcription factor Egr1, induces expression of a 67-kDa form of glutamic acid decarboxylase in embryonic hippocampal neurons. J Biol Chem 2008; 283:24789–24800Crossref, Medline, Google Scholar
18 : Activity-dependent expression of the transcription factor Zif268 reveals ocular dominance columns in monkey visual cortex. Brain Res 1993; 605:349–353Crossref, Medline, Google Scholar
19 : Immediate early gene expression in cat visual cortex during and after the critical period: differences between EGR-1 and Fos proteins. Brain Res Mol Brain Res 1996; 36:12–22Crossref, Medline, Google Scholar
20 : Layer-specific differential regulation of transcription factors Zif268 and Jun-D in visual cortex V1 and V2 of macaque monkeys. Neuroscience 1997; 81:653–666Crossref, Medline, Google Scholar
21 : High basal expression of the zif/268 immediate early gene in cortical layers IV and VI, in CA1 and in the corpus striatum: an in situ hybridization study. Neurosci Lett 1991; 122:67–70Crossref, Medline, Google Scholar
22 : Constitutive expression of zif268 in neocortex is regulated by synaptic activity. Proc Natl Acad Sci USA 1991; 88:5106–5110Crossref, Medline, Google Scholar
23 : Distribution of immediate early gene zif-268, c-fos, c-jun, and jun-D mRNAs in the adult cat with special references to brain region related to vision. Neurosci Lett 1994; 176:137–141Crossref, Medline, Google Scholar
24 : A combined analysis of microarray gene expression studies of the human prefrontal cortex identifies genes implicated in schizophrenia. J Psychiatr Res 2012; 46:1464–1474Crossref, Medline, Google Scholar
25 : Genetic analysis of the calcineurin pathway identifies members of the EGR gene family, specifically EGR3, as potential susceptibility candidates in schizophrenia. Proc Natl Acad Sci USA 2007; 104:2815–2820Crossref, Medline, Google Scholar
26 : Induction of immediate-early genes and the control of neurotransmitter-regulated gene expression within the nervous system. Pharmacol Rev 1995; 47:133–178Medline, Google Scholar
27 : Deficits in transcriptional regulators of cortical parvalbumin neurons in schizophrenia. Am J Psychiatry 2012; 169:1082–1091Link, Google Scholar
28 : Conserved regional patterns of GABA-related transcript expression in the neocortex of subjects with schizophrenia. Am J Psychiatry 2008; 165:479–489Link, Google Scholar
29 : Lower gene expression for KCNS3 potassium channel subunit in parvalbumin-containing neurons in the prefrontal cortex in schizophrenia. Am J Psychiatry 2014; 171:62–71Link, Google Scholar
30 : Gene expression deficits in a subclass of GABA neurons in the prefrontal cortex of subjects with schizophrenia. J Neurosci 2003; 23:6315–6326Crossref, Medline, Google Scholar
31 : The influence of chronic exposure to antipsychotic medications on brain size before and after tissue fixation: a comparison of haloperidol and olanzapine in macaque monkeys. Neuropsychopharmacology 2005; 30:1649–1661Crossref, Medline, Google Scholar
32 : Allelic variation in GAD1 (GAD67) is associated with schizophrenia and influences cortical function and gene expression. Mol Psychiatry 2007; 12:854–869Crossref, Medline, Google Scholar
33 : Prefrontal dysfunction in schizophrenia involves mixed-lineage leukemia 1-regulated histone methylation at GABAergic gene promoters. J Neurosci 2007; 27:11254–11262Crossref, Medline, Google Scholar
34 : Developmental pattern of perineuronal nets in the human prefrontal cortex and their deficit in schizophrenia. Biol Psychiatry 2013; 74:427–435Crossref, Medline, Google Scholar
35 : Extracellular matrix-glial abnormalities in the amygdala and entorhinal cortex of subjects diagnosed with schizophrenia. Arch Gen Psychiatry 2010; 67:155–166Crossref, Medline, Google Scholar
36 : How necessary is the activation of the immediate early gene zif268 in synaptic plasticity and learning? Behav Brain Res 2003; 142:17–30Crossref, Medline, Google Scholar
37 : Local and descending circuits regulate long-term potentiation and zif268 expression in spinal neurons. Eur J Neurosci 2006; 24:761–772Crossref, Medline, Google Scholar
38 : Deep brain stimulation of the mediodorsal thalamic nucleus yields increases in the expression of zif-268 but not c-fos in the frontal cortex. J Chem Neuroanat 2013; 52:20–24Crossref, Medline, Google Scholar
39 : Reduced dendritic spine density on cerebral cortical pyramidal neurons in schizophrenia. J Neurol Neurosurg Psychiatry 1998; 65:446–453Crossref, Medline, Google Scholar
40 : Decreased dendritic spine density on prefrontal cortical pyramidal neurons in schizophrenia. Arch Gen Psychiatry 2000; 57:65–73Crossref, Medline, Google Scholar
41 : Dendritic spine pathology in schizophrenia. Neuroscience 2013; 251:90–107Crossref, Medline, Google Scholar
42 : Patterns of intrinsic and associational circuitry in monkey prefrontal cortex. J Comp Neurol 1996; 376:614–630Crossref, Medline, Google Scholar