Quantitative Morphology of the Caudate and Putamen in Patients With Cocaine Dependence
Abstract
OBJECTIVE: Deficits in dopaminergic function may contribute to hypertrophy of striatal structures associated with typical neuroleptic treatment. In light of a body of research that has associated chronic cocaine use with extrapyramidal symptoms and striatal dopaminergic depletion, the authors looked for evidence of striatal dysmorphology in patients with chronic cocaine dependence. METHOD: Caudate, putamen, and total brain volumes were quantified by means of magnetic resonance imaging in 25 cocaine-dependent and 20 healthy subjects. RESULTS: Normalized caudate and putamen volumes were 3.40% and 9.18% larger, respectively, in the cocaine-dependent subjects. CONCLUSIONS: These observations suggest that deficits in dopaminergic function associated with cocaine dependence may contribute to striatal hypertrophy.
Persistent blockade of dopamine transporters by chronic cocaine abuse may lead to depletion of brain dopamine levels (1), possibly as a result of this blockade rendering neurons unable to reuptake and recycle dopamine, which is instead metabolized in the synapse and excreted (2). Depletion of dopamine in the putamen may contribute to the increased frequency of extrapyramidal symptoms (including tics, choreoathetoid movements, resting hand tremor, and dystonia [3–6]) observed in cocaine-dependent patients (1, 7). Treatment with neuroleptic medications that block dopamine 2 (D2) receptors also decreases striatal dopaminergic neurotransmission, increases the frequency of extrapyramidal symptoms (8), and is associated with striatal enlargement. This enlargement normalizes after patients are switched to atypical antipsychotic medications, which have lower affinities for the D2 receptor (9).
Given the greater frequency of extrapyramidal symptoms in cocaine abusers, the evidence that chronic exposure to cocaine depletes striatal dopamine, and the association of striatal enlargement with neuroleptic blockade of D2 receptors, we hypothesized that caudate and putamen volumes would be larger in subjects with cocaine dependence. Given the role of the putamen in motor control and the higher concentration of dopamine transporters in the putamen relative to the caudate (10), we anticipated that this effect would be greater for the putamen.
Method
Healthy and cocaine-dependent subjects were administered the SCID (11) and underwent a physical and neurologic examination and routine laboratory testing that included a urine toxicology screening analysis. Twenty-five subjects (10 women and 15 men; 14 African American and 11 Caucasian; mean age=35.6 years, SD=5.7, range=20–45) who met criteria for chronic cocaine dependence without a comorbid axis I disorder participated. On average, these subjects had smoked 4.5 g (SD=5.9) of crack cocaine per week for 15.3 years (SD=6.5) and consumed 3.7 (SD=5.2) alcoholic drinks per day, typically in the context of cocaine use. One cocaine-dependent subject also met criteria for alcohol dependence. Subjects with an abuse or dependence history with any other class of drug were excluded. Cocaine-dependent subjects all had urine toxicology results that were positive for the cocaine metabolite benzoylecgonine upon study admission; subjects were scanned 15.3 days (SD=12.0) after their last cocaine use.
The 20 healthy comparison subjects (11 women and nine men; five African American and 15 Caucasian; mean age=35.0 years, SD=6.8, range=22–48) participating were free of any axis I disorder and consumed 0.3 (SD=0.7) alcoholic drinks per day. All subjects were free of current or previous psychotropic medication exposure. Twenty-four of the cocaine-dependent subjects and 17 of the healthy subjects were right-handed.
All subjects provided written informed consent for participation in this study, which was approved by the Yale and VA Human Investigations Committees.
All subjects were scanned with the same 1.5-T Signa (GE Medical Systems, Milwaukee) magnetic resonance scanner. Volumes of the total brain, caudate, and putamen were measured from a coronal series acquired by using three-dimensional spoiled gradient recalled echo in the steady state (acquisition matrix=256 × 192, number of excitations=1, flip angle=45°, TE=5 msec, TR=24 msec, field of view=24 cm2, slice thickness=1.9 mm [slice thickness=3 mm for 10 healthy and four cocaine-dependent subjects]).
All scans were reoriented in MEDx (Sensor Systems, Sterling, Va.) such that the axial plane was parallel to the intercommissural plane. Quantitative measurements of brain morphology were then performed in ANALYZE (Mayo Foundation, Rochester, Minn.) by a rater blind to diagnosis (L.K.J.). Volumes were calculated by multiplying area measurements by slice thickness. The caudate, including head and body, and putamen were quantified by manually outlining these structures on each coronal slice in which they were visible (12). Total brain volume was measured by thresholding images to exclude ventricular cerebrospinal fluid and then manually outlining brain tissue, including the pons and cerebellum, on sequential coronal slices. Ten brains measured twice by the same rater revealed intrarater reliabilities (intraclass correlation coefficients [ICCs] [13]) of 0.98 for the left and right caudate, 0.95 for the left putamen, and 0.97 for the right putamen. Measurement of five brains by the same rater indicated an intrarater ICC of 0.99 for total brain volume.
Group differences in demographic variables and total brain volume were assessed by using chi-square analyses and t tests for independent groups. Group differences in caudate and putamen morphology were assessed by dividing the volume of each structure by the corresponding total brain volume to obtain a measure of volume proportional to total brain volume and performing repeated measures analysis of covariance (ANCOVA), with diagnosis as a between-subjects variable, side as a within-subjects variable, and age and gender as covariates. The relationship between age and caudate and putamen volumes was examined by using partial correlation analysis that controlled for diagnosis and gender. Within the cocaine-dependent group, the relationships between caudate and putamen volumes and both reported cocaine use in the past 6 months (bags per week) and time since last cocaine use were also examined.
Results
Cocaine-dependent and healthy subjects did not differ in age, handedness, gender, number of tobacco cigarettes smoked per day, or total brain volume (cocaine-dependent subjects: mean=1359.5 cm3, SD=126.8; healthy subjects: mean=1385.0 cm3, SD=166.0). Healthy subjects had two more years of education (mean=14.4 years [SD=2.0] versus mean=12.4 years, [SD=2.1]; t=3.43, df=43, p=0.001) and reported lower alcohol intake than did the cocaine-dependent subjects (t=2.85, df=43, p=0.007). The ratio of African American to Caucasian subjects was greater among cocaine-dependent subjects than among healthy subjects (χ2=4.38, df=1, p=0.04). Age was significantly inversely correlated with right and left caudate volume (r=–0.42, df=41, p=0.004, and r=–0.52, df=41, p=0.0003, respectively) and right and left putamen volume (r=–0.40, df=41, p=0.008, and r=–0.40, df=41, p=0.008).
An ANCOVA of the caudate/total brain volume ratio and the putamen/total brain volume ratio that controlled for age and gender revealed a significant effect of diagnosis (Figure 1). In the cocaine-dependent subjects, normalized caudate volumes were 4.33% larger on the left and 2.46% larger on the right relative to those of the healthy comparison subjects (left: mean=2.65 [SD=0.18] versus 2.54 [SD=0.33], respectively; right: mean=2.91 [SD=0.20] versus 2.84 [SD=0.31]). Normalized putamen volumes in the cocaine-dependent subjects were also 9.77% larger on the left and 8.59% larger on the right relative to those of the healthy comparison subjects (left: mean=2.92 [SD=0.36] versus 2.66 [SD=0.30], respectively; right: mean=3.16 [SD=0.32] versus 2.91 [SD=0.34]). The right-greater-than-left effect of side was significant for the putamen but not for the caudate (Figure 1). There were no significant interactions. When amount of daily alcohol use was entered as a third covariate into the ANCOVA, the effect of cocaine dependence on caudate volume was no longer significant (F=3.23, df=1, 40, p=0.08), whereas the effect on putamen volume remained significant (F=6.44, df=1, 40, p=0.02). Similarly, when race was entered as a third covariate, the effect of cocaine dependence on caudate volume was no longer significant (F=3.65, df=1, 40, p=0.06), whereas the effect on putamen volume remained significant (F=5.19, df=1, 40, p=0.03). Reported cocaine use over the past 6 months and time since last cocaine use were not significantly correlated with caudate or putamen volumes.
Discussion
In this study, evidence of caudate and putamen enlargement was observed in a group of patients with chronic cocaine dependence. The degree of enlargement was greater for the putamen, consistent with its role in motor control and extrapyramidal symptoms and with the greater concentration of dopamine transporters in the putamen relative to the caudate (1, 7, 10).
A mediating factor in the enlargement of the putamen and caudate in subjects with chronic cocaine dependence may be the depletion of striatal dopamine and consequent decreases in striatal dopaminergic neurotransmission. Evidence for depletion of striatal dopamine following chronic exposure to cocaine has been observed in both in vivo (14) and postmortem (1) studies of human chronic cocaine abusers and in some (15–17) but not all (18) studies of rats chronically exposed to cocaine. Discrepancies in some of the preclinical data likely reflect differences between species or patterns and methods of cocaine administration. The hypothesis that the relative striatal enlargement in these patients may stem from cocaine-induced depletion of striatal dopamine is consistent with the elevated frequency of extrapyramidal symptoms observed in the cocaine-dependent patients and the enlarged striatal volumes in patients receiving neuroleptics that block D2 receptors and reduce striatal dopaminergic neurotransmission (9). However, other potential etiologies of this enlargement, such as drug-induced increases in perfusion of the striatum (9), cannot be excluded by these data. The well-documented deficits in cerebral blood flow associated with chronic cocaine use argue against this latter interpretation (19).
The right-greater-than-left asymmetry observed for the putamen and the age-related decline in the volumes of both the putamen and caudate are consistent with previous observations in healthy subjects (20). Limitations of this study include the acquisition of slightly thicker slices in 10 healthy and four cocaine-dependent subjects, which may have contributed additional variance to the morphometric measures. In addition, since extrapyramidal symptoms were not measured in these subjects, the relationship between degree of extrapyramidal symptoms and degree of striatal enlargement could not be examined. Cocaine-dependent subjects consumed significantly greater amounts of alcohol than did healthy subjects, which is a reflection of the common co-use of alcohol with cocaine (21). When this difference was controlled for, the effect of cocaine dependence on normalized putamen volumes remained significant, whereas this effect on normalized caudate volumes was no longer significant. Similarly, when the difference in racial composition across diagnostic groups was controlled for, the effect of cocaine dependence on normalized putamen volumes remained significant, whereas this effect on normalized caudate volumes was no longer significant. Our failure to observe a relationship between amount or recency of cocaine use and volume of the caudate or putamen may reflect the relative inaccuracy of self-report data. Last, these data cannot address the important question of whether the observed enlargement of striatal structures is reversible, as it appears to be when due to neuroleptic exposure (9). Answering such a question will require longitudinal follow-up of patients with documented abstinence from cocaine.
Received April 28, 2000; revision received Sept. 11, 2000; accepted Sept. 22, 2000. From the Department of Psychiatry, Yale University School of Medicine, New Haven, Conn., and the VA Connecticut Healthcare System. Reprints for this article are not available. Address correspondence to Dr. Jacobsen, Psychiatry 116A, VA Connecticut Healthcare System, 950 Campbell Ave., West Haven, CT 06516; [email protected] (e-mail). Supported in part by National Institute on Drug Abuse grants DA-00167, DA-04060, and DA-09250 and by the U.S. Department of Veterans Affairs (the VA Connecticut-Massachusetts Mental Illness Research, Education and Clinical Center). The authors thank Jonathan S. Feinstein, Ph.D., for statistical consultation and Quinn Ramsby for computer support.
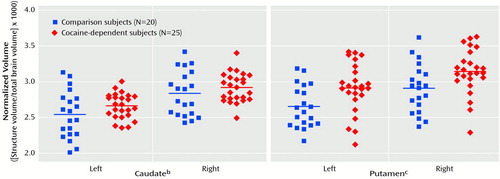
Figure 1. Differences in Caudate and Putamen Morphology Between Cocaine-Dependent and Healthy Comparison Subjectsa
aMorphological differences assessed by dividing volume of each structure by the total brain volume. Horizontal lines represent means.
bSignificant effect of diagnosis (F=4.23, df=1, 41, p<0.05).
cSignificant effect of diagnosis (F=7.55, df=1, 41, p=0.009) and side (F=7.02, df=1, 41, p=0.01).
1. Wilson JM, Levey AI, Bergeron C, Kalasinsky K, Ang L, Peretti F, Adams VI, Smialek J, Anderson WR, Shannak K, Deck J, Niznik HB, Kish SJ: Striatal dopamine, dopamine transporter, and vesicular monoamine transporter in chronic cocaine users. Ann Neurol 1996; 40:428–439Crossref, Medline, Google Scholar
2. Dackis C, Gold MS: Neurotransmitter and neuroendocrine abnormalities associated with cocaine use. Psychiatr Med 1987; 3:461–483Google Scholar
3. Bartzokis G, Beckson M, Wirshing DA, Lu PH, Foster JA, Mintz J: Choreoathetoid movements in cocaine dependence. Biol Psychiatry 1999; 45:1630–1635Google Scholar
4. Bauer LO: Resting hand tremor in abstinent cocaine-dependent, alcohol-dependent, and polydrug-dependent patients. Alcohol Clin Exp Res 1996; 20:1196–1201Google Scholar
5. Fines RE, Brady WJ, DeBehnke DJ: Cocaine-associated dystonic reaction. Am J Emerg Med 1997; 15:513–516Crossref, Medline, Google Scholar
6. Attig E, Amyot R, Botez T: Cocaine induced chronic tics. J Neurol Neurosurg Psychiatry 1994; 57:1143–1144Google Scholar
7. Alexander GE, DeLong MR, Strick PL: Parallel organization of functionally segregated circuits linking basal ganglia and cortex. Annu Rev Neurosci 1986; 9:357–381Crossref, Medline, Google Scholar
8. Baldessarini RJ: Drugs and the treatment of psychiatric disorders: psychosis and anxiety, in Goodman and Gilman’s The Pharmacological Basis of Therapeutics. Edited by Hardman JG, Limbird LE, Molinoff PB, Ruddon RW. New York, McGraw-Hill, 1996, pp 399–430Google Scholar
9. Corson PW, Nopoulos P, Miller DD, Arndt S, Andreasen NC: Change in basal ganglia volume over 2 years in patients with schizophrenia: typical versus atypical neuroleptics. Am J Psychiatry 1999; 156:1200–1204Google Scholar
10. Donnan GA, Kaczmarczyk SJ, Paxinos G, Chilco PJ, Kalnins RM, Woodhouse DG, Mendelsohn FAO: Distribution of catecholamine uptake sites in human brain as determined by quantitative [3H] mazindol autoradiography. J Comp Neurol 1991; 304:419–434Crossref, Medline, Google Scholar
11. First MB, Spitzer RL, Gibbon M, Williams JBW: Structured Clinical Interview for DSM-IV Axis I Disorders (SCID). New York, New York State Psychiatric Institute, Biometrics Research, 1997Google Scholar
12. Giedd JN, Snell JW, Lange N, Rajapakse JC, Casey BJ, Kozuch PL, Vaituzis AC, Vauss YC, Hamburger SD, Kaysen D, Rapoport JL: Quantitative magnetic resonance imaging of human brain development: ages 4–18. Cereb Cortex 1996; 6:551–560Crossref, Medline, Google Scholar
13. Fleiss JL: The Design and Analysis of Clinical Experiments. New York, John Wiley & Sons, 1986Google Scholar
14. Volkow ND, Wang GJ, Fowler JS, Logan J, Gatley SJ, Hitzemann R, Chen AD, Dewey SL, Pappas N: Decreased striatal dopaminergic responsiveness in detoxified cocaine-dependent subjects. Nature 1997; 386:830–833Crossref, Medline, Google Scholar
15. Wilson JM, Nobrega JN, Carroll ME, Niznik HB, Shannak K, Lac ST, Pristupa ZB, Dixon LM, Kish SJ: Heterogenous subregional binding patterns of 3H-WIN 35,428 and 3H-GBR 12,935 are differentially regulated by chronic cocaine self-administration. J Neurosci 1994; 14:2966–2979Google Scholar
16. Maisonneuve IM, Ho A, Kreek MJ: Chronic administration of a cocaine “binge” alters basal extracellular levels in male rats: an in vivo microdialysis study. J Pharmacol Exp Ther 1995; 272:652–657Medline, Google Scholar
17. Howard SG, Fisher R, Landry CF: Alterations in the spontaneous release of dopamine and the density of the DA D2 receptor mRNA after chronic postnatal exposure to cocaine. Brain Res Bull 1997; 43:101–106Crossref, Medline, Google Scholar
18. Jones SR, Lee TH, Wightman RM, Ellinwood EH: Effects of intermittent and continuous cocaine administration on dopamine release and uptake regulation in the striatum: in vitro voltammetric assessment. Psychopharmacology (Berl) 1996; 126:331–338Crossref, Medline, Google Scholar
19. Volkow ND, Mullani N, Gould KL, Adler S, Krajewski K: Cerebral blood flow in chronic cocaine users: a study with positron emission tomography. Br J Psychiatry 1988; 152:641–648Crossref, Medline, Google Scholar
20. Gunning-Dixon FM, Head D, McQuain J, Acker JD, Raz N: Differential aging of the human striatum: a prospective MR imaging study. AJNR Am J Neuroradiol 1998; 19:1501–1507Google Scholar
21. Gorelick DA: Alcohol and cocaine: clinical and pharmacological interactions. Recent Dev Alcohol 1992; 10:37–56Crossref, Medline, Google Scholar