The Effect of Paroxetine on 5-HT2A Receptors in Depression: An [18F]Setoperone PET Imaging Study
Abstract
OBJECTIVE: In the cortex of animals, serotonin (5-HT) levels increase after several weeks of treatment with selective serotonin reuptake inhibitors (SSRIs). Studies using an intrasubject design to examine the effects of SSRI treatment on 5-HT2A receptors in the cortex of drug-free depressed patients are needed. In theory, agonist stimulation of 5-HT2A receptors could be relevant to SSRI treatment by promoting neuronal growth and survival as well as direct elevation of mood. The objective of this study was to evaluate the effect of 6 weeks of paroxetine treatment on 5-HT2A receptors in depressed patients. METHOD: After a medication-free period of at least 3 months, 19 depressed patients were treated for 6 weeks with paroxetine, 20 mg/day. The authors used [18F]setoperone and positron emission tomography to assess 5-HT2A receptor binding potential in the patients before and after treatment and in 19 age-matched healthy subjects. RESULTS: 5-HT2A binding potential declined with age in all cortical regions in the depressed and healthy subjects. There was a significant interaction between age and treatment effect on 5-HT2A binding potential in all cortical regions. Subjects aged 20 to 30 years had a 10% decrease in 5-HT2A binding potential after treatment, whereas subjects aged 30 to 40 had no change. No regional differences in 5-HT2A binding potential between depressed and healthy subjects were found. CONCLUSIONS: 5-HT2A receptors down-regulate in young depressed subjects after treatment with paroxetine, but this down-regulation attenuates with age. This suggests that over 6 weeks paroxetine treatment increases 5-HT agonism on 5-HT2A receptors in the cortex of young patients with depression.
While the specific mechanisms of antidepressant treatment are not known, serotonergic neurons may be important because they are targeted by selective serotonin reuptake inhibitors (SSRIs). In animal models, long-term SSRI administration raises intrasynaptic serotonin (5-HT) levels in the cortex (1). This could affect different types of 5-HT receptors, but there are several reasons why 5-HT2A receptors could contribute to SSRI antidepressant effects. First, 5-HT2A receptors have the potential to activate cAMP-response element-binding protein and/or brain-derived neurotrophic factor through Ca2+-dependent protein kinases in the cortex (2, 3). It is possible that elevated 5-HT concentrations after SSRI treatment could elevate mood by means of 5-HT2A receptors because drugs with 5-HT2A agonist properties may have euphoriant effects (4–8). The euphoria can occur after administration of low doses of 2,5-dimethoxy-4-methyl-amphetamine when hallucinations are not present (5, 7, 8).
While in theory some SSRI effects could be mediated by 5-HT2A receptors, it is not known whether SSRIs at clinical doses have any effect at all on 5-HT2A receptors in humans. Preclinical data with long-term SSRI treatment are not consistent, although there is a tendency toward up-regulation or no change at lower doses (9–11) and down-regulation at higher doses (12, 13). Rats have much faster drug metabolism than humans, and it may be that intraperitoneal dosing of SSRIs in rats differs from oral dosing in humans. We are aware of one published study of humans. Using [18F]setoperone positron emission tomography (PET), Massou et al. (14) found that a group of six depressed patients responding to SSRI treatment had higher 5-HT2A receptor binding potential than a separate group of eight depressed patients who were untreated. This study did not use an intrasubject design, and five of the six treated patients were also taking benzodiazepines. It is reported that benzodiazepines lower serotonin release in animals, and this presents a potential confound (15–17).
To our knowledge, the relationship between 5-HT2A receptors during depression and subsequent response to treatment has not been investigated. Postmortem methods cannot be used, and we know of no imaging study of 5-HT2A receptors that has examined this question. Platelet 5-HT2A receptor Bmax (receptor density) is another in vivo measurement. Platelet and frontal cortex 5-HT2A receptors have identical structures; hence, platelet measures may be used as a peripheral index of cortex 5-HT2A receptors (18). One such study (19) showed no association between platelet 5-HT2A Bmax before treatment and the eventual response to SSRIs.
[18F]Setoperone has several properties favorable for PET imaging of 5-HT2A receptors in the human cortex (20). Setoperone is an antagonist with high affinity and specificity for 5-HT2A receptors in the cortex (6). The affinity of setoperone is 100 times as great for 5-HT2A receptors as for 5-HT2C receptors (6), and cortex 5-HT2C receptor density is relatively low (21, 22). The affinity of setoperone for 5-HT2A receptors is 10 to 50 times as great as its affinity for D2 receptors, but D2 receptors have a low density in the cortex (23). Both 5-HT2A,C and D2 antagonists displace [18F]setoperone in the striatum, but only 5-HT2A,C antagonists displace [18F]setoperone in the cortex; thus, specific binding of [18F]setoperone in the cortex reflects binding to 5-HT2A receptors. 5-HT2A antagonists do not displace [18F]setoperone in the cerebellum, and the cerebellum is almost devoid of 5-HT2A receptors, hence it may be used as a reference region (24, 25). Metabolites of [18F]setoperone do not show significant brain uptake (26). Other properties have been previously reviewed (27).
The main purpose of this study was to use [18F]setoperone PET to investigate the effect of 6 weeks of treatment with clinical doses of paroxetine on 5-HT2A receptors in depressed patients. A secondary aim was to assess whether 5-HT2A binding potential is abnormal in treatment-responsive patients before treatment.
Since SSRIs raise intrasynaptic 5-HT levels in animals (1) and 5-HT2A agonists down-regulate 5-HT2A receptors (28), it was hypothesized that with clinical doses of paroxetine, down-regulation of 5-HT2A receptors would occur. We also hypothesized that 5-HT2A receptor binding potential would not differ in treatment responders given the report that platelet 5-HT2A Bmax in drug-free depressed patients showed no relationship with later SSRI response (19).
Method
Subjects
This study was approved by the University of Toronto Human Subjects Review Committee. Nineteen depressed subjects aged 18 to 41 years and 19 age-matched healthy subjects aged 18 to 41 years were recruited by advertisement. All subjects were free of psychotropic drug use for more than 3 months. Data on the prefrontal cortex from baseline (pretreatment) scans of 14 depressed subjects and 19 healthy subjects have been previously reported (27). All of the subjects were physically healthy and right-handed. For each subject, written consent was obtained after the procedures had been fully explained.
The healthy subjects were screened by using the Structured Clinical Interview for DSM-IV—Non-Patient Edition (29). A diagnosis of a major depressive episode secondary to major depressive disorder was confirmed by the Structured Clinical Interview for DSM-IV Axis I Disorders, Patient Edition (SCID-P) (30), which was administered by a trained research assistant. Each patient received a psychiatric consultation (by J.H.M. or S.H.K.) to verify the SCID-P diagnosis. A score of greater than 17 on the 17-item Hamilton Depression Rating Scale (31) was required for entry into the study. Patients with psychotic symptoms, bipolar disorder (type I or II), or comorbid axis I diagnoses were excluded, as were subjects with a history of alcohol or drug abuse or dependence. In addition, all past drug use was recorded. Subjects with a history of substance use that did not meet the criteria for the SCID-P diagnosis of substance abuse received a urine drug screen and then were included in the study only if the result was negative. Six subjects had previously completed an antidepressant trial longer than 6 weeks, but none had received any antidepressant treatment within the last 6 months. Two had a history of past suicide attempts. Each depressed subject had routine tests to rule out common medical causes of depression (thyroid function, electrolyte levels, complete blood cell count).
Imaging and Treatment Protocol
For the depressed subjects, [18F]setoperone scanning occurred before and after treatment. The treatment was paroxetine, 20 mg/day in the evening for 6 weeks. Before treatment, the patients were informed that their serum paroxetine levels would be sampled on the day of the second [18F]setoperone PET scan. The serum sample and second [18F]setoperone PET scan took place 11 to 15 hours after the last paroxetine dose, when the intersubject variability of serum paroxetine levels is low. The samples taken were frozen at –20°C and subsequently assayed by means of high-performance liquid chromatography with fluorescence detection (Medical Toxicology Unit, Guy’s & St. Thomas’ Hospital Trust, London, U.K.) (32).
Treatment response was determined by the Hamilton depression score at the end of 6 weeks of treatment. A final score of 16 or greater indicated nonresponse. A score of 9 to 15 indicated a partial response, and a score of 8 or less was considered a full response.
[18F]Setoperone was prepared by [18F]fluoride substitution on the nitro-derivative precursor of setoperone (33). The [18F]setoperone was of high radiochemical purity (>99%) and high specific activity (mean=51 GBq/μmol, SD=36, at the time of injection). Imaging was based on the approach described by Blin et al. (34). An intravenous bolus of 185 MBq of [18F]setoperone was injected. PET images were obtained by using a GEMS 2048-15B camera. Images were obtained in five 1-minute frames, followed by 17 5-minute frames. The images were corrected for attenuation by using a 68Ge transmission scan and reconstructed by filtered back projection (Hanning filter, 5 mm full width at half maximum).
Data Analysis
To obtain a measure of 5-HT2A binding potential, we used the pseudoequilibrium cortex-to-cerebellum ratio minus one (35). In a two-tissue compartment model, one compartment is composed of free and nonspecifically bound ligand, and the second compartment represents setoperone specifically bound to 5-HT2A receptors. Given that the cerebellum has no displaceable [18F]setoperone binding, the data for the time activity curve within this region represent the first tissue compartment of free and nonspecifically bound ligand (20, 24). With the pseudoequilibrium ratio method, if the free and nonspecifically bound ligand within the cerebellum is representative of the free and nonspecifically bound ligand in the cortex, then the ratio (cortex–cerebellum)/cerebellum is proportional to k3/k4 as well as Bmax/Kd. (k3 and k4 are rate constants for ligand transfer between the two tissue compartments. Bmax represents the receptor density, and Kd is the dissociation constant for the ligand at the receptor. Pseudoequilibrium refers to a period of time in which the k3/k4 ratio is stable.) Between 65 and 90 minutes, pseudoequilibrium is present, as there is no significant change in the cortex-to-cerebellum ratio (35). Petit-Taboue et al. (36) empirically demonstrated that the pseudoequilibrium cortex-to-cerebellar ratio is highly correlated with the kinetically obtained k3/k4 ratio (r=0.91–0.97). Between 0 and 90 minutes, under the condition of high doses of 5-HT2A antagonists, it has been demonstrated that the parameters describing the first tissue compartment (free and nonspecific) are similar in the cerebellum and cortex (24). By using the cortex-to-cerebellum ratio, the need for an arterial puncture is avoided, making this approach less invasive.
Traditionally, binding potential values are determined within regions of interest. There are a couple of limitations to region-of-interest analyses. They do not describe the spatial extent of a finding. If the region of interest does not overlap the spatial extent of a true difference, power may decline. Voxel-based analyses may offer greater sensitivity under such circumstances.
In the present study, two methods were used; one was a voxel-by-voxel analysis with statistical parametric mapping (37–39), and the second was a region-of-interest approach. For the first method, parametric binding potential images were created by using the pseudoequilibrium cortex-to-cerebellum ratio. Then these individual images were transformed and deformed to obtain a common brain shape within Montreal Neurological Institute space by using the ligand-specific template method developed for SPM 96 (40). Then the images were spatially smoothed with a Gaussian filter (12 mm full width at half maximum).
The statistical analysis was done with SPM 99. With the transformed and deformed PET images of 5-HT2A binding potential, statistical parametric mapping generates an analysis at each voxel that assigns a p value to each voxel, resulting in an image of probability values. In this study the threshold for display within the probability image was set at p<0.05. The voxels displayed do not account for multiple comparisons. Given the known distributions of random probability values in three-dimensional space, statistical parametric mapping can correct for multiple comparisons by assessing the number of adjacent suprathreshold voxels (cluster significance) or by setting extremely low individual voxel value thresholds (peak significance) (39). To avoid false positives, a conservative approach was used: the regions displayed were considered only if the change was significant for corrected cluster significance or corrected peak significance (p<0.05). All peak height and cluster thresholds reported are corrected for multiple-voxel comparisons and are not based on any a priori hypotheses with respect to the regional location.
For the region-of-interest method, each subject had a magnetic resonance imaging (MRI) scan (GE Signa 1.5-T scanner, spin-echo sequence proton-density-weighted image; x, y, z voxel dimensions 0.78, 0.78, 3.00 mm, respectively). The MRI scans were coregistered to each PET image (41). For region-of-interest analysis, the cerebellum regions of interest were drawn on summated [18F]setoperone scans with reference to the coregistered MRI scan by a rater blinded to the identity of the scans. The nine cortex regions of interest were found as follows: points in Montreal Neurological Institute template space were found in the center of the medial frontal gyrus (Brodmann’s area 9), lateral orbitofrontal cortex, parahippocampal gyrus, posteromedial temporal gyrus, and rostral anterior cingulate. Each point was found bilaterally except the rostral anterior cingulate. These points were transformed onto the original PET summated image by using the inverse of the 12-parameter component of the statistical parametric mapping spatial normalization. For each point now on the original image, the nearest 75 to 300 voxels were chosen above an edge threshold (depending on region). See Table 1 for the region locations and sizes. Then the regions of interest were automatically superimposed on the PET image and individually visually verified to be accurate by means of the coregistered MRI. Decay-corrected time activity curves were obtained for each of these regions of interest.
The technique just described is well standardized in our laboratory. To test the reliability of this measure, seven normal subjects were scanned twice, and their data showed a test-retest standard deviation of 8% and a mean scan-rescan difference of 6%. We have found that this technique is sensitive to age-related changes in 5-HT2A receptors (27, 35, 42).
Results
Nineteen patients (12 male, seven female; mean age=30.8 years, SD=6.1) were scanned before and after treatment. The mean baseline score on the Hamilton Depression Rating Scale of these patients was 21.8 (SD=3.8). All patients scanned before and after treatment had detectable serum paroxetine levels and were included in the analysis (mean=30 μg/liter, SD=24).
Nineteen age-matched healthy subjects were recruited (eight male, 11 female; mean age=31.8, SD=6.9); 18 were matched within 1 year of age, and one was matched within 2 years. There was no significant difference in gender between groups (Fisher’s exact test, p=0.33).
In the following sections we will first report results of our reassessment, in a larger study group, of previous findings: the age-associated decline in 5-HT2A binding potential (27, 43–45) and the lack of diagnosis effect on 5-HT2A binding potential (27). Then the primary question of a treatment effect will be addressed by examining the effect of treatment on cortical 5-HT2A binding potential and the effect of the interaction between age and treatment on cortical 5-HT2A binding potential. Finally, we will examine whether initial cortical 5-HT2A binding potential has any association with eventual treatment response.
Effects of Age
Since age has been previously associated with a decline in both 5-HT2A Bmax and 5-HT2A binding potential (27, 43–45), we assessed the effect of age on 5-HT2A binding potential. In the healthy subjects, age was a significant covariate globally in the cortex in both type of analyses. In the voxel-wise analysis, analysis of covariance (ANCOVA) at each voxel (86,821 voxels) yielded corrected probability levels of p<0.001 (corrected peak) and p<0.001 (corrected cluster). Similar levels resulted from the ANCOVAs for the regions of interest (F=19–30, df=1, 17, p<0.001).
Within the pretreatment scans, all cortical regions showed an association with age (Figure 1); for the ANCOVAs at each voxel (80,688 voxels), p<0.001 (corrected peak) and p<0.001 (corrected cluster). Within the posttreatment scans, all cortical regions also showed an association with age; for the ANCOVAs at each voxel (80,477 voxels), p<0.001 (corrected peak) and p<0.001 (corrected cluster). Then the relationship between age and 5-HT2A binding potential was examined in each region within the pretreatment scans and then within the posttreatment scans. Every region showed a significant association with age (ANCOVA, F=15–30, df=1, 17, p<0.001).
Effects of Diagnosis
While the main aim of this study was to examine the effects of paroxetine treatment in depression, the treatment effect is better appreciated in the context of how 5-HT2A binding potential may differ between depressed and healthy subjects. The scans of the patients before treatment were compared to the scans of the healthy subjects by using ANCOVA with age as a covariate; a similar comparison was conducted with the patients’ posttreatment scans. With the voxel-by-voxel approach, no differences in 5-HT2A binding potential between the healthy subjects and depressed patients (pretreatment or posttreatment scans) were found. Similarly, no differences in 5-HT2A binding potential were found between the depressed and healthy subjects in the region-of-interest analyses. Furthermore, differences in 5-HT2A binding potential between the two groups did not interact with age in the voxel-wise or region-of-interest analyses.
Effects of Treatment
5-HT2A binding potential within a large region composed of bilateral occipital and medial temporal cortex decreased with treatment, but this was not significant when the cluster threshold was applied; for the repeated measures ANCOVA with age as a covariate (3,377 voxels), p=0.48 (corrected peak) and p=0.20 (corrected cluster). The results of region-of-interest analyses of 5-HT2A binding potential using repeated measures (within subject) ANCOVA were not significant. Having a full response (N=10) versus having a partial response or nonresponse (N=9) had no association with change in 5-HT2A binding potential with treatment in voxel-wise or regional analyses. Using the region-of-interest data, we examined a plot of the residual values versus age, and an obvious decrease with treatment was present in younger individuals.
Interaction Between Treatment Effect and Age
The effect of the interaction between treatment and age on 5-HT2A binding potential was found globally in the voxel-by-voxel analysis; for the repeated measures ANCOVA of the treatment-by-age interaction (56,928 voxels), p<0.05 (corrected peak) and p<0.001 (corrected cluster) (Figure 2). The decrease in 5-HT2A binding potential after treatment became less pronounced as age increased. This finding was also examined with the region-of-interest approach. Using multivariate repeated measures analysis of covariance (MANCOVA), we found the effect of treatment, the treatment-by-age interaction, and the effect of region were significant with respect to 5-HT2A binding potential (treatment: F=7.71, df=1, 17, p=0.01; treatment by age: F=6.33, df=1, 17, p=0.02; region: F=2.92, df=8, 10, p=0.05). The multiple variables refer to the different regions, and the repeated measure is the within-subject value before and after treatment. Repeated measures ANCOVA with age as a covariate for each region showed that the treatment-by-age interaction was significant for almost every region (Table 2). The within-subject repeated measure is the region-of-interest 5-HT2A binding potential. In patients aged 20 to 30 years, the mean decrease was 10% across all regions, whereas in patients aged 30 to 40 the mean change was 0%. For the region-of-interest analysis, there were no outliers, as the Cook’s distances were less than 1 for all observations. Pearson correlation indicated that there was no correlation between age and serum paroxetine level (r=–0.02, N=19, p=0.93).
Relation of Treatment Response to Pretreatment 5-HT2A Binding Potential
The depressed patients were classified according to treatment response, resulting in 10 treatment responders, six partial responders, and three nonresponders. No differences in 5-HT2A binding potential were found between the responders and the remaining patients (partial responders and nonresponders) in either the voxel-based or regional analyses using ANCOVA to examine the effect of being an eventual responder, with age as a covariate. No interaction between age and difference in 5-HT2A binding potential between groups was present. The age of the treatment responders matched that of the non- and partial responders within 2 years, with the exception of an additional 21-year-old subject in the treatment-responding group.
5-HT2A binding potential in the 10 treatment-responding subjects was compared to 5-HT2A binding potential in the age-matched healthy subjects. The voxel-wise analysis showed globally higher 5-HT2A binding potential in the treatment-responding patients; for the ANCOVA of the diagnosis effect in the responders (44,852 voxels), p<0.18 (corrected peak) and p<0.001 (corrected cluster). A region-of-interest MANCOVA showed somewhat greater 5-HT2A binding potential in the treatment-responding patients than in the healthy subjects (diagnosis: F=2.62, df=9, 9, p=0.09; age: F=5.63, df=9, 9, p=0.009). The multiple variables in the MANCOVA were the different regions. Within individual regions, there was no significant difference between the treatment-responding and healthy subjects.
5-HT2A binding potential in the remaining nine subjects—the partial responders and nonresponding patients—was compared to that in a different set of nine age-matched healthy subjects. Using ANCOVA with voxel-based analyses and MANCOVA and ANCOVA for the region-of-interest data, we found no differences between these two groups (and no age interaction was present).
Discussion
To our knowledge, this is the first report of 5-HT2A receptor changes during SSRI treatment shown by a within-subject design in previously drug-free depressed patients. In patients under age 30, we found decreases of 5-HT2A binding potential in all brain regions; however, these decreases attenuated with increasing age.
There are two alternative explanations for the decrease in 5-HT2A binding potential with paroxetine treatment. One is that paroxetine increased 5-HT secondary to 5-HT transporter blockade (1) and that 5-HT2A down-regulation occurred. The second is that paroxetine directly antagonized 5-HT2A receptors and decreased the detected 5-HT2A receptor binding potential. This latter possibility can be ruled out. Using [18F]setoperone PET, we previously demonstrated no change in 5-HT2A receptor binding potential after single-dose paroxetine administration, thus ruling out a short-term antagonist effect in vivo (46). Moreover, the affinity of paroxetine for 5-HT2A receptors is extremely low, being in the nonspecific range (6). On the other hand, the first explanation is plausible since long-term SSRI exposure is associated with increased synaptic 5-HT (1).
The binding potential reflects Bmax/Kd, and hence the down-regulation observed could be attributed to either decreased Bmax (decreased receptor density) or increased Kd (decreased affinity). In ex vivo studies, changes in 5-HT2A receptors after long-term agonist administration are characterized mostly by decreased 5-HT2A Bmax(47). Thus, it is likely that decreased 5-HT2A Bmax would account for the majority of the change in 5-HT2A binding potential found in the current study.
The decrease in 5-HT2A binding potential after treatment became less so with age. It could be that down-regulation of 5-HT2A receptors decreases with age. This notion is consistent with the well-established finding that 5-HT2A receptor density decreases with age (27, 43–45), since a floor effect would limit down-regulation with age. To our knowledge, the relationship between age and down-regulation of 5-HT2A receptors has not been investigated in animal models, but some aspects of 5-HT2A receptor regulation appear to change with age. One effector pathway of 5-HT2A receptors includes activation of phospholipase C, production of inositol triphosphate, increased intracellular calcium, and activation of protein kinase C (48, 49). Activation of protein kinase C influences stability of messenger RNA for 5-HT2A receptors, and the activation of calcium-dependent protein kinase C has been reported to change with age in rodent brains (50).
Our finding contrasts with that of Massou et al. (14), who reported higher 5-HT2A receptor binding potential in six treated depressed patients than in eight untreated depressed patients. There are several methodological differences between this study and the current one that could explain the discrepancy. The earlier study did not use an intrasubject design, the dose and type of SSRI varied, concomitant benzodiazepines were used, and the timing of the [18F]setoperone scan varied among subjects. Benzodiazepines may influence 5-HT release (15–17). The timing of the second scan relative to the onset of treatment could influence results because preclinical models of SSRI administration demonstrate that both the firing rate of cortical 5-HT neurons and the concentration of 5-HT may fluctuate markedly over the course of treatment (1, 51, 52).
It has been reported that 5-HT2A binding potential declines after treatment with desipramine (53). The authors of that study proposed that decreases in 5-HT2A binding potential might contribute to a mechanism of therapeutic effect for desipramine. With SSRI treatment it seems unlikely that down-regulation itself is directly therapeutic because in the current study there was no association between down-regulation and treatment response. On the other hand, the power to find this association may be very low for several reasons. The true difference could be small. Placebo responders add variance. The degree of down-regulation could vary among individuals of similar age because of genetic differences, adding more variance. 5-HT agonism on multiple receptors rather than one receptor may be important for therapeutic effect; thus, down-regulation of a single receptor subtype may not associate strongly with treatment response.
Even if down-regulation itself is not a therapeutic effect of SSRIs, this does not rule out the interesting possibility that increased 5-HT agonism on 5-HT2A receptors is therapeutic. While down-regulation of 5-HT2A receptors may occur in the presence of increased 5-HT, down-regulation itself can reduce the agonist effect of 5-HT on 5-HT2A receptors.
The decrease in 5-HT2A binding potential with treatment in younger subjects was a global cortical change. Given that 5-HT transporter sites are widespread throughout the cortex, it is not surprising that paroxetine would have global effects on 5-HT2A receptors (54).
We found no difference between depressed and healthy subjects in any cortical region. This is consistent with findings from studies of medication-free depressed subjects (27, 45).
It is likely that 5-HT reuptake inhibition resulted in down-regulation of the 5-HT2A binding potential. In theory, indirect mechanisms, such as occupancy by endogenous 5-HT, could also contribute to a decrease in 5-HT2A receptor binding potential. The concentration of endogenous 5-HT in the human cortex is about 0.5 to 1.5 nM (55), and the KI of 5-HT for cortical 5-HT2A receptors is 300 to 5000 nM (6) (KI is the concentration at which one-half of the receptor sites are displaced by a drug or chemical and is inversely proportional to the affinity of the drug or chemical). If these cortical 5-HT concentrations reflect synaptic concentrations, about 1% of synaptic 5-HT2A receptors could be occupied by 5-HT at baseline. This is consistent with a finding in our previous study (46), in which we found that single-dose paroxetine had no effect on the setoperone binding potential of healthy subjects.
Several methodological limitations are present in the study. The resolution of the PET camera does not allow one to distinguish the cell type or cellular location attributable to the change in 5-HT2A receptors. Candidate cell types likely to account for a large proportion of 5-HT2A receptors would include interneurons as well as pyramidal cell neurons (56). Another limitation is that the binding potential reflects Bmax/Kd, and we were unable to discern between these two measures. However, down-regulation processes are the predominant responses to agonist stimulation in ex vivo models (47). With respect to the paroxetine treatment, we took many precautions to enhance compliance, such as informing patients that their serum levels were to be assayed at the time of the second PET scan as part of the study. We also took measurements and detected paroxetine levels in every patient. Even so, it is possible that some patients may have temporarily interrupted their treatment earlier, and this would not be detected in our protocol.
In conclusion, we found a down-regulation of 5-HT2A receptor binding potential after paroxetine treatment in younger depressed patients. This suggests that long-term paroxetine treatment increases 5-HT agonism on 5-HT2A receptors in the cortex of young patients with depression.
![]() |
![]() |
Received March 20, 2000; revision received July 14, 2000; accepted July 19, 2000. From the Clarke Division, Centre for Addictions and Mental Health, Department of Psychiatry, University of Toronto. Address reprint requests to Dr. Meyer, Clarke Division, Centre for Addictions and Mental Health, Department of Psychiatry, University of Toronto, 250 College St., Toronto, Ont., Canada M5T 1R8; [email protected] (e-mail). Supported by Medical Research Council of Canada grant MT-14337, by Canadian Psychiatric Research Foundation grant 8764, and by the National Alliance for Research in Schizophrenia and Depression. The authors thank research assistants Corey Jones, Alex Kecojevic, and Verdell Golding and technicians Doug Hussey, Kevin Cheung, Armando Garcia, and Li Jin.
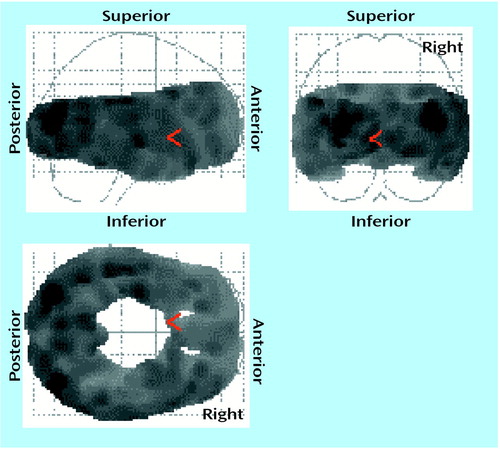
Figure 1. Global Cortical Decline in 5-HT2A Binding Potential With Age in 19 Depressed Patientsa
ap<0.05 for each voxel. Results of analysis of covariance were converted into z scores and then probability values. Degrees of freedom for individual voxels=1, 17, the number of voxels was 80,688, and p<0.001 was the corrected cluster significance. Age negatively covaried with 5-HT2A binding potential in all regions.
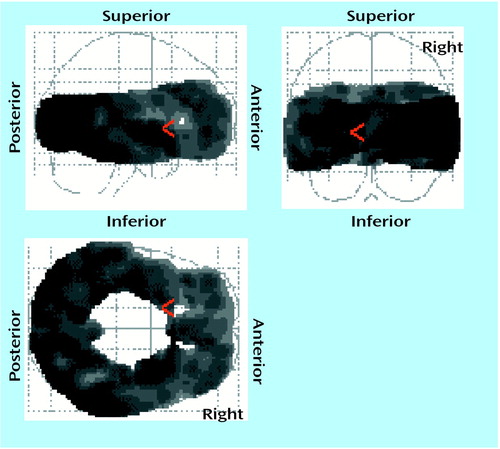
Figure 2. Age-Related Attenuation of Decrease in Cortical 5-HT2A Binding Potential After Paroxetine Treatment in 19 Depressed Patientsa
ap<0.05 for each voxel. Results of repeated measures analysis of covariance examining interaction of treatment effect with age were converted into z scores and then probability values. Degrees of freedom for individual voxels=1, 34, the number of voxels was 56,928, and p<0.001 was the corrected cluster significance. At each voxel the decrease after treatment interacted with age such that the decrease after treatment became less with age.
1. Bel N, Artigas F: Chronic treatment with fluvoxamine increases extracellular serotonin in frontal cortex but not in raphe nuclei. Synapse 1993; 15:243–245Crossref, Medline, Google Scholar
2. Duman R, Heninger G, Nestler E: A molecular and cellular theory of depression. Arch Gen Psychiatry 1997; 54:597–606Crossref, Medline, Google Scholar
3. Vaidya V, Marek G, Aghajanian G, Duman R:5-HT2A receptor-mediated regulation of brain derived neurotrophic factor mRNA in the hippocampus and the neocortex. J Neurosci 1997; 17:2785–2795Google Scholar
4. Newton RA, Phipps SL, Flanigan TP, Newberry NR, Carey JE, Kumar C, McDonald B, Chen C, Elliott JM: Characterisation of human 5-hydroxytryptamine2A and 5-hydroxytryptamine2C receptors expressed in the human neuroblastoma cell line SH-SY5Y: comparative stimulation by hallucinogenic drugs. J Neurochem 1996; 67:2521–2531Google Scholar
5. Hollister L, Macnicol M, Gillespie H: An hallucinogenic amphetamine analog (DOM) in man. Psychopharmacology (Berl) 1969; 14:62–73Crossref, Google Scholar
6. Seeman P: Receptor Tables, Vol 2: Drug Dissociation Constants for Neuroreceptors and Transporters. Toronto, Schizophrenia Research, 1993, section 26Google Scholar
7. Snyder SH, Faillace L, Hollister L:2,5-Dimethoxy-4-methyl-amphetamine (STP): a new hallucinogenic drug. Science 1967; 158:669–670Google Scholar
8. Snyder SH, Faillace LA, Weingartner H: DOM (STP), a new hallucinogenic drug, and DOET: effects in normal subjects. Am J Psychiatry 1968; 125:357–364Link, Google Scholar
9. Peroutka SJ, Snyder SH: Long-term antidepressant treatment decreases spiroperidol-labeled serotonin receptor binding. Science 1980; 210:88–90Crossref, Medline, Google Scholar
10. Dumbrille-Ross A, Tang S: Manipulations of synaptic serotonin: discrepancy of effects on serotonin S1 and S2 sites. Life Sci 1983; 32:2677–2684Google Scholar
11. Hrdina P, Vu T: Chronic fluoxetine treatment upregulates 5-HT uptake sites and 5-HT2 receptors in rat brain: an autoradiographic study. Synapse 1993; 14:324–331Crossref, Medline, Google Scholar
12. Paul I, Duncan G, Powell K, Mueller R, Hong J, Breese G: Regionally specific adaptation of beta adrenergic and 5-hydroxytryptamine2 receptors. J Pharmacol Exp Ther 1988; 246:956–962Medline, Google Scholar
13. Wamsley JK, Byerley WF, McCabe RT, McConnell EJ, Dawson TM, Grosser BI: Receptor alterations associated with serotonergic agents: an autoradiographic analysis. J Clin Psychiatry 1987; 48(March suppl):19–25Google Scholar
14. Massou J, Trichard C, Attar-Levy D, Feline A, Corruble E, Beaufils B, Martinot J: Frontal 5-HT2A receptors studied in depressive patients during chronic treatment by selective serotonin reuptake inhibitors. Psychopharmacology (Berl) 1997; 133:99–101Crossref, Medline, Google Scholar
15. Yoshioka M, Matsumoto M, Togashi H, Saito H: Effects of conditioned fear stress on 5-HT release in the rat prefrontal cortex. Pharmacol Biochem Behav 1995; 51:515–519Crossref, Medline, Google Scholar
16. Tao R, Ma Z, Auerbach SB: Differential regulation of 5-hydroxytryptamine release by GABAA and GABAB receptors in midbrain raphe nuclei and forebrain of rats. Br J Pharmacol 1996; 119:1375–1384Google Scholar
17. Pei Q, Zetterstrom T, Fillenz M: Both systemic and local administration of benzodiazepine agonists inhibit the in vivo release of 5-HT from ventral hippocampus. Neuropharmacology 1989; 28:1061–1066Google Scholar
18. Cook EJ, Fletcher K, Wainwright M, Marks N, Yan S, Leventhal B: Primary structure of the human platelet serotonin 5-HT2A receptor: identity with the frontal cortex serotonin 5-HT2A receptor. J Neurochem 1994; 63:465–469Crossref, Medline, Google Scholar
19. Hrdina P, Bakish D, Ravindran A, Chudzik J, Cavazzoni P, Lapierre Y: Platelet serotonergic indices in major depression: up-regulation of 5-HT2A receptors unchanged by antidepressant treatment. Psychiatry Res 1997; 66:73–85Crossref, Medline, Google Scholar
20. Blin J, Pappata S, Kiyosawa M, Crouzel C, Baron J: [18F]Setoperone: a new high-affinity ligand for positron emission tomography study of the serotonin-2 receptors in baboon brain in vivo. Eur J Pharmacol 1988; 147:73–82Crossref, Medline, Google Scholar
21. Gross-Isseroff R, Salama D, Israeli M, Biegon A: Autoradiographic analysis of [3H]ketanserin binding in the human brain postmortem: effect of suicide. Brain Res 1990; 507:208–215Crossref, Medline, Google Scholar
22. Marazziti D, Rossi A, Giannaccini G, Zavaglia KM, Dell’Osso L, Lucacchini A, Cassano GB: Distribution and characterization of [3H]mesulergine binding in human brain postmortem. Eur Neuropsychopharmacol 1999; 10:21–26Crossref, Medline, Google Scholar
23. De Keyser J, Clayes A, DeBacker J-P, Ebinger G, Roels F, Vauqelin G: Autoradiographic localization of D1 and D2 dopamine receptors in the human brain. Neurosci Lett 1988; 91:142–147Crossref, Medline, Google Scholar
24. Fischman A, Bonab A, Babich J, Alpert N, Rauch S, Elmaleh D, Shoup T, Williams S, Rubin R: Positron emission tomographic analysis of central 5-hydroxytryptamine2 receptor occupancy in healthy volunteers treated with the novel antipsychotic agent ziprasidone. J Pharmacol Exp Ther 1996; 279:939–947Medline, Google Scholar
25. Schotte A, Maloteaux J, Laduron P: Characterization and regional distribution of serotonin S2-receptors in human brain. Brain Res 1983; 276:231–235Crossref, Medline, Google Scholar
26. Blin J, Crouzel C: Blood-cerebrospinal fluid and blood-brain barriers imaged by 18F-labeled metabolites of 18F-setoperone studied in humans using positron emission tomography. J Neurochem 1992; 58:2303–2310Google Scholar
27. Meyer JH, Kapur S, Houle S, DaSilva J, Owczarek B, Brown GM, Wilson AA, Kennedy SH: Prefrontal cortex 5-HT2 receptors in depression: an [18F]setoperone PET imaging study. Am J Psychiatry 1999; 156:1029–1034Google Scholar
28. Leysen JE, Janssen PF, Niemegeers CJ: Rapid desensitization and down-regulation of 5-HT2 receptors by DOM treatment. Eur J Pharmacol 1989; 163:145–149Crossref, Medline, Google Scholar
29. Spitzer RL, Williams JBW, Gibbon M, First MB: Structured Clinical Interview for DSM-IV—Non-Patient Edition (SCID-NP, Version 1.0). Washington, DC, American Psychiatric Press, 1995Google Scholar
30. First MB, Spitzer RL, Gibbon M, Williams JBW: Structured Clinical Interview for DSM-IV Axis I Disorders, Patient Edition (SCID-P), version 2. New York, New York State Psychiatric Institute, Biometrics Research, 1995Google Scholar
31. Hamilton M: A rating scale for depression. J Neurol Neurosurg Psychiatry 1960; 23:56–62Crossref, Medline, Google Scholar
32. Spencer E: An improved method for the measurement of paroxetine in human/serum plasma by HPLC with fluorescence detection (abstract). Ther Drug Monit 1999; 21:483Crossref, Google Scholar
33. Maziere B, Crouzel C, Venet M, Stulzaft O, Sanz G, Ottaviani M, Sejourne C, Pascal O, Bisserbe J: Synthesis, affinity and specificity of 18F-setoperone, a potential ligand for in-vivo imaging of cortical serotonin receptors. Nucl Med Biol 1988; 15:463–468Google Scholar
34. Blin J, Sette G, Fiorelli M, Bletry O, Elghozi JL, Crouzel C, Baron JC: A method for the in vivo investigation of the serotonergic 5-HT2 receptors in the human cerebral cortex using positron emission tomography and 18F-labeled setoperone. J Neurochem 1990; 54:1744–1754Google Scholar
35. Kapur S, Jones C, DaSilva J, Wilson A, Houle S: Reliability of a simple non-invasive method for the evaluation of 5-HT2 receptors using [18F]setoperone PET imaging. Nucl Med Commun 1997; 18:395–399Crossref, Medline, Google Scholar
36. Petit-Taboue MC, Landeau B, Osmont A, Tillet I, Barre L, Baron JC: Estimation of neocortical serotonin-2 receptor binding potential by single-dose fluorine-18-setoperone kinetic PET data analysis. J Nucl Med 1996; 37:95–104Medline, Google Scholar
37. Friston K, Ashburner J, Frith C, Poline J-B, Heather J, Frackowiak R: Spatial registration and normalisation of images. Hum Brain Mapp 1995; 2:165–189Crossref, Google Scholar
38. Friston K, Holmes A, Worsley K, Poline J, Frith C, Frackowiak R: Statistical parametric maps in functional imaging: a general linear approach. Hum Brain Mapp 1995; 2:189–210Crossref, Google Scholar
39. Friston K, Frith C, Liddle P, Frackowiak R: Assessing the significance of focal activations using their spatial extent. Hum Brain Mapp 1994; 1:214–220Google Scholar
40. Meyer JH, Gunn RN, Myers R, Grasby PM: Assessment of spatial normalization of PET ligand images using ligand-specific templates. Neuroimage 1999; 9:545–553Crossref, Medline, Google Scholar
41. Studholme C, Hill DL, Hawkes DJ: Automated three-dimensional registration of magnetic resonance and positron emission tomography brain images by multiresolution optimization of voxel similarity measures. Med Phys 1997; 24:25–35Crossref, Medline, Google Scholar
42. Lewis R, Kapur S, Jones C, DaSilva J, Brown GM, Wilson AA, Houle S, Zipursky RB: Serotonin 5-HT2 receptors in schizophrenia: a PET study using [18F]setoperone in neuroleptic-naive patients and normal subjects. Am J Psychiatry 1999; 156:72–78Link, Google Scholar
43. Arranz B, Eriksson A, Mellerup E, Plenge P, Marcusson J: Effect of aging in human cortical pre- and postsynaptic serotonin binding sites. Brain Res 1993; 620:163–166Crossref, Medline, Google Scholar
44. Gross-Isseroff R, Salama D, Israeli M, Biegon A: Autoradiographic analysis of [3H]ketanserin binding in the human brain postmortem: effect of suicide. Brain Res 1990; 507:208–215Crossref, Medline, Google Scholar
45. Meltzer CC, Price JC, Mathis CA, Greer PJ, Cantwell MN, Houck PR, Mulsant BH, Ben-Eliezer D, Lopresti B, DeKosky ST, Reynolds CF III: PET imaging of serotonin type 2A receptors in late-life neuropsychiatric disorders. Am J Psychiatry 1999; 156:1871–1878Google Scholar
46. Meyer JH, Cho R, Kennedy S, Kapur S: The effects of single dose nefazodone and paroxetine upon 5-HT2A binding potential in humans using [18F]-setoperone PET. Psychopharmacology (Berl) 1999; 144:279–281Crossref, Medline, Google Scholar
47. Leysen J, Pauwels P:5-HT2 receptors, roles and regulation. Ann NY Acad Sci 1990; 600:183–191Google Scholar
48. Berg K, Maayani S, Clarke W: Interactions between effectors linked to serotonin receptors. Ann NY Acad Sci 1998; 861:111–120Crossref, Medline, Google Scholar
49. Roth B, Willins D, Kristiansen K, Kroeze W:5-Hydroxytryptamine2–family receptors (5-hydroxytryptamine2A, 5-hydroxytryptamine2B, 5-hydroxytryptamine2C): where structure meets function. Pharmacol Ther 1998; 79:231–257Google Scholar
50. Pascale A, Govoni S, Battaini F: Age-related alteration of PKC, a key enzyme in memory processes: physiological and pathological examples. Mol Neurobiol 1998; 16:49–62Crossref, Medline, Google Scholar
51. Bel N, Artigas F: Fluvoxamine preferentially increases extracellular 5-hydroxytryptamine in the raphe nuclei: an in vivo microdialysis study. Eur J Pharmacol 1992; 229:101–103Crossref, Medline, Google Scholar
52. Blier P, De Montigny C: Electrophysiological investigations on the effect of repeated zimelidine administration on serotonergic neurotransmission in the rat. J Neurosci 1983; 3:1270–1278Google Scholar
53. Yatham LN, Liddle PF, Dennie J, Shiah IS, Adam MJ, Lane CJ, Lam RW, Ruth TJ: Decrease in brain serotonin2 receptor binding in patients with major depression following desipramine treatment: a positron emission tomography study with fluorine-18-labeled setoperone. Arch Gen Psychiatry 1999; 56:705–711Crossref, Medline, Google Scholar
54. Laruelle M, Vanisberg M-A, Maloteaux J-M: Regional and subcellular localization in human brain of [3H]paroxetine binding, a marker of serotonin uptake sites. Biol Psychiatry 1988; 24:299–309Crossref, Medline, Google Scholar
55. Azmitia E, Gannon P: The primate serotonergic system: a review of human and animal studies and a report on Macaca fascicularis. Adv Neurol 1986; 43:407–468Medline, Google Scholar
56. Jakab R, Goldman-Rakic P:5-Hydroxytryptamine2A serotonin receptors in the primate cerebral cortex: possible site of action of hallucinogenic and antipsychotic drugs in pyramidal cell apical dendrites. Proc Natl Acad Sci USA 1998; 95:735–740Google Scholar